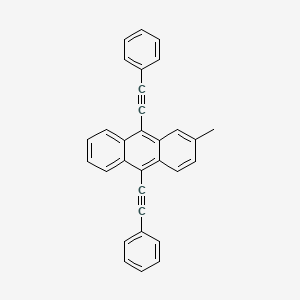
9,10-Bis(phenylethynyl)-2-methylanthracene
Descripción general
Descripción
9,10-Bis(phenylethynyl)-2-methylanthracene is a polycyclic aromatic hydrocarbon known for its unique photophysical properties. This compound is characterized by its high quantum yield and stability, making it a valuable material in various scientific and industrial applications .
Métodos De Preparación
Synthetic Routes and Reaction Conditions
The synthesis of 9,10-Bis(phenylethynyl)-2-methylanthracene typically involves the Sonogashira cross-coupling reaction. This method uses palladium catalysts to couple phenylethynyl groups to the anthracene core . The reaction conditions often include the use of copper iodide as a co-catalyst and an amine base in an inert atmosphere, such as nitrogen or argon, to prevent oxidation .
Industrial Production Methods
While specific industrial production methods for this compound are not widely documented, the general approach involves scaling up the laboratory synthesis methods. This includes optimizing reaction conditions to increase yield and purity while ensuring cost-effectiveness and safety in an industrial setting .
Análisis De Reacciones Químicas
Types of Reactions
9,10-Bis(phenylethynyl)-2-methylanthracene undergoes various chemical reactions, including:
Oxidation: This reaction can lead to the formation of quinones or other oxygenated derivatives.
Reduction: Reduction reactions can convert the compound into more saturated derivatives.
Substitution: Electrophilic substitution reactions can introduce different functional groups onto the anthracene core.
Common Reagents and Conditions
Oxidation: Common oxidizing agents include potassium permanganate and chromium trioxide.
Reduction: Reducing agents such as lithium aluminum hydride or sodium borohydride are often used.
Substitution: Reagents like halogens (chlorine, bromine) and nitrating agents (nitric acid) are commonly employed.
Major Products Formed
The major products formed from these reactions depend on the specific reagents and conditions used. For example, oxidation can yield anthraquinones, while substitution reactions can produce halogenated or nitrated anthracenes .
Aplicaciones Científicas De Investigación
9,10-Bis(phenylethynyl)-2-methylanthracene has a wide range of applications in scientific research:
Mecanismo De Acción
The mechanism of action of 9,10-Bis(phenylethynyl)-2-methylanthracene involves its ability to absorb and emit light. Upon excitation by light, the compound undergoes a transition to an excited state, followed by the emission of light as it returns to the ground state. This process is influenced by the molecular structure and the surrounding environment, such as solvent viscosity and temperature .
Comparación Con Compuestos Similares
Similar Compounds
9,10-Bis(phenylethynyl)anthracene: Similar in structure but lacks the methyl group at the 2-position.
9-(4-Phenylethynyl)anthracene: Contains only one phenylethynyl group.
9-(4-Phenyl)anthracene: Contains a phenyl group instead of a phenylethynyl group.
Uniqueness
9,10-Bis(phenylethynyl)-2-methylanthracene is unique due to the presence of the methyl group at the 2-position, which can influence its photophysical properties and reactivity. This structural modification can lead to differences in fluorescence efficiency and stability compared to its analogs .
Propiedades
IUPAC Name |
2-methyl-9,10-bis(2-phenylethynyl)anthracene | |
---|---|---|
Source | PubChem | |
URL | https://pubchem.ncbi.nlm.nih.gov | |
Description | Data deposited in or computed by PubChem | |
InChI |
InChI=1S/C31H20/c1-23-16-19-29-28(20-17-24-10-4-2-5-11-24)26-14-8-9-15-27(26)30(31(29)22-23)21-18-25-12-6-3-7-13-25/h2-16,19,22H,1H3 | |
Source | PubChem | |
URL | https://pubchem.ncbi.nlm.nih.gov | |
Description | Data deposited in or computed by PubChem | |
InChI Key |
KBIWITFMKHHGQY-UHFFFAOYSA-N | |
Source | PubChem | |
URL | https://pubchem.ncbi.nlm.nih.gov | |
Description | Data deposited in or computed by PubChem | |
Canonical SMILES |
CC1=CC2=C(C3=CC=CC=C3C(=C2C=C1)C#CC4=CC=CC=C4)C#CC5=CC=CC=C5 | |
Source | PubChem | |
URL | https://pubchem.ncbi.nlm.nih.gov | |
Description | Data deposited in or computed by PubChem | |
Molecular Formula |
C31H20 | |
Source | PubChem | |
URL | https://pubchem.ncbi.nlm.nih.gov | |
Description | Data deposited in or computed by PubChem | |
DSSTOX Substance ID |
DTXSID70542664 | |
Record name | 2-Methyl-9,10-bis(phenylethynyl)anthracene | |
Source | EPA DSSTox | |
URL | https://comptox.epa.gov/dashboard/DTXSID70542664 | |
Description | DSSTox provides a high quality public chemistry resource for supporting improved predictive toxicology. | |
Molecular Weight |
392.5 g/mol | |
Source | PubChem | |
URL | https://pubchem.ncbi.nlm.nih.gov | |
Description | Data deposited in or computed by PubChem | |
CAS No. |
51580-23-5 | |
Record name | 2-Methyl-9,10-bis(phenylethynyl)anthracene | |
Source | EPA DSSTox | |
URL | https://comptox.epa.gov/dashboard/DTXSID70542664 | |
Description | DSSTox provides a high quality public chemistry resource for supporting improved predictive toxicology. | |
Retrosynthesis Analysis
AI-Powered Synthesis Planning: Our tool employs the Template_relevance Pistachio, Template_relevance Bkms_metabolic, Template_relevance Pistachio_ringbreaker, Template_relevance Reaxys, Template_relevance Reaxys_biocatalysis model, leveraging a vast database of chemical reactions to predict feasible synthetic routes.
One-Step Synthesis Focus: Specifically designed for one-step synthesis, it provides concise and direct routes for your target compounds, streamlining the synthesis process.
Accurate Predictions: Utilizing the extensive PISTACHIO, BKMS_METABOLIC, PISTACHIO_RINGBREAKER, REAXYS, REAXYS_BIOCATALYSIS database, our tool offers high-accuracy predictions, reflecting the latest in chemical research and data.
Strategy Settings
Precursor scoring | Relevance Heuristic |
---|---|
Min. plausibility | 0.01 |
Model | Template_relevance |
Template Set | Pistachio/Bkms_metabolic/Pistachio_ringbreaker/Reaxys/Reaxys_biocatalysis |
Top-N result to add to graph | 6 |
Feasible Synthetic Routes
Descargo de responsabilidad e información sobre productos de investigación in vitro
Tenga en cuenta que todos los artículos e información de productos presentados en BenchChem están destinados únicamente con fines informativos. Los productos disponibles para la compra en BenchChem están diseñados específicamente para estudios in vitro, que se realizan fuera de organismos vivos. Los estudios in vitro, derivados del término latino "in vidrio", involucran experimentos realizados en entornos de laboratorio controlados utilizando células o tejidos. Es importante tener en cuenta que estos productos no se clasifican como medicamentos y no han recibido la aprobación de la FDA para la prevención, tratamiento o cura de ninguna condición médica, dolencia o enfermedad. Debemos enfatizar que cualquier forma de introducción corporal de estos productos en humanos o animales está estrictamente prohibida por ley. Es esencial adherirse a estas pautas para garantizar el cumplimiento de los estándares legales y éticos en la investigación y experimentación.