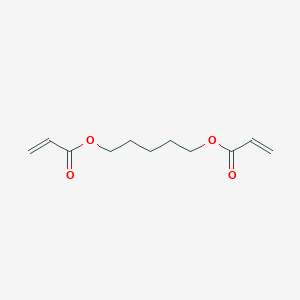
1,5-Pentanediol diacrylate
Descripción general
Descripción
1,5-Pentanediol diacrylate is a multifunctional acrylic monomer used primarily in the production of UV and electron beam curable coatings, adhesives, and plastics. This compound is derived from 1,5-pentanediol, a diol that serves as a building block for various chemical syntheses. The unique structure of this compound allows it to enhance the properties of the materials it is incorporated into, such as increasing crosslink density and reducing viscosity .
Aplicaciones Científicas De Investigación
1,5-Pentanediol diacrylate has numerous applications in scientific research and industry:
Coatings: It is used in UV and electron beam curable coatings for wood, plastics, and metals, providing enhanced durability and resistance to environmental factors
Adhesives: The compound is incorporated into adhesives to improve their bonding strength and flexibility.
Biomedical Applications: Research is ongoing into the use of this compound in biomedical applications, such as drug delivery systems and tissue engineering.
Mecanismo De Acción
Target of Action
1,5-Pentanediol diacrylate (PDDA) is a multifunctional acrylic monomer primarily used in UV and EB cure formulations . Its primary targets are the components of these formulations, where it serves to reduce viscosity and increase crosslink density .
Mode of Action
The mode of action of PDDA involves its interaction with other compounds in the adhesive or coating formulation. The acrylate groups in PDDA react with these compounds, resulting in a durable and strong bond . This interaction leads to enhanced film formation and improved performance of the coating .
Biochemical Pathways
PDDA is synthesized from 1,5-pentanediol (1,5-PDO), which is made from furfural, a chemical derived from renewable hemicellulose (biomass) sources such as wood and crop wastes . The synthesis of PDDA from 1,5-PDO represents a significant biochemical pathway . In addition, 1,5-PDO can be produced by hydrogenation of glutaric acid and its derivatives .
Result of Action
The result of PDDA’s action is the formation of a strong, durable bond in adhesive or coating formulations . It enhances film formation and improves the performance of the coating . In UV and EB cure formulations, PDDA produces nearly identical properties compared to hexanediol diacrylate (HDDA) in most formulations, with opportunities for improved performance in ink jet and adhesives formulations .
Action Environment
The action of PDDA is influenced by the environmental conditions of the UV or EB cure formulation process. Factors such as the presence of other compounds in the formulation, the intensity of UV or EB radiation, and the temperature can all influence the efficacy and stability of PDDA . Furthermore, PDDA is a bio-based material derived from renewable sources, which means its production has a minimum impact on the environment .
Análisis Bioquímico
Cellular Effects
As a multifunctional acrylic monomer, PDDA likely influences cell function in the context of its use in UV and EB cure formulations . This could include impacts on cell signaling pathways, gene expression, and cellular metabolism.
Molecular Mechanism
It’s known that PDDA can exert its effects at the molecular level, potentially through binding interactions with biomolecules, enzyme inhibition or activation, and changes in gene expression
Transport and Distribution
Given its use in UV and EB cure formulations, it’s likely that PDDA interacts with certain transporters or binding proteins
Métodos De Preparación
1,5-Pentanediol diacrylate can be synthesized through the esterification of 1,5-pentanediol with acrylic acid. This reaction typically requires a catalyst, such as sulfuric acid or p-toluenesulfonic acid, to proceed efficiently. The reaction conditions often involve heating the mixture to a temperature range of 60-80°C and removing the water formed during the reaction to drive the equilibrium towards the formation of the diacrylate ester .
In industrial settings, this compound can also be produced from renewable sources. For example, 1,5-pentanediol can be derived from furfural, a chemical obtained from biomass sources like wood and crop wastes. This bio-based route involves the hydrogenation of furfural to produce 1,5-pentanediol, which is then esterified with acrylic acid to form this compound .
Análisis De Reacciones Químicas
1,5-Pentanediol diacrylate undergoes various chemical reactions, including:
Polymerization: This compound can undergo free radical polymerization in the presence of initiators like benzoyl peroxide or azobisisobutyronitrile (AIBN).
Esterification: As mentioned earlier, this compound is formed through the esterification of 1,5-pentanediol with acrylic acid.
Comparación Con Compuestos Similares
1,5-Pentanediol diacrylate is often compared to other diacrylate compounds, such as hexanediol diacrylate and butanediol diacrylate. While all these compounds serve similar functions in coatings, adhesives, and plastics, this compound offers unique advantages:
Lower Viscosity: Compared to hexanediol diacrylate, this compound has a lower viscosity, making it easier to process and incorporate into formulations
Bio-based Production: Unlike some other diacrylates, this compound can be produced from renewable sources, offering a more sustainable alternative
Similar compounds include:
- Hexanediol diacrylate
- Butanediol diacrylate
- Ethylene glycol diacrylate
- Trimethylolpropane triacrylate
These compounds share similar functionalities but differ in their specific properties and applications.
Actividad Biológica
1,5-Pentanediol diacrylate (PDDA) is a difunctional acrylate monomer synthesized from renewable sources, primarily used in UV-curable coatings and adhesives. Its biological activity is increasingly relevant due to its potential applications in biomedical fields, including drug delivery systems and tissue engineering. This article provides a comprehensive overview of the biological activity of PDDA, supported by data tables, case studies, and detailed research findings.
This compound is characterized by the following chemical properties:
Property | Value |
---|---|
Molecular Formula | C11H16O4 |
Molecular Weight | 212.24 g/mol |
Boiling Point | 90-95 °C (at 0.1 Torr) |
Density | 1.024 ± 0.06 g/cm³ |
Solubility | Slightly soluble in chloroform, soluble in ethyl acetate |
PDDA is synthesized from 1,5-pentanediol (PDO), which can be derived from renewable biomass through a multi-step process involving furfural as a starting material . This bio-based approach not only reduces reliance on petroleum but also offers a more sustainable alternative to traditional monomers like 1,6-hexanediol diacrylate (HDDA).
Cytotoxicity and Biocompatibility
Studies have demonstrated that PDDA exhibits low cytotoxicity compared to other acrylate monomers. For instance, in a study assessing the cytotoxic effects of various acrylates on human dermal fibroblasts, PDDA showed significantly lower toxicity levels than HDDA . This makes it a suitable candidate for applications in biomedicine where biocompatibility is crucial.
Drug Delivery Systems
PDDA has been investigated for its potential use in drug delivery systems due to its ability to form hydrogels. These hydrogels can encapsulate therapeutic agents and provide controlled release profiles. In vitro studies indicate that PDDA-based hydrogels can effectively release drugs over extended periods while maintaining structural integrity .
Gene Delivery Applications
Research has explored the use of PDDA in gene delivery applications. A study involving nanoparticles made from PDDA demonstrated enhanced transfection efficiency compared to traditional liposomal systems. The lower cytotoxicity of PDDA nanoparticles allows for higher doses of nucleic acids to be delivered without significant cell damage . This characteristic positions PDDA as a promising vector for non-viral gene therapy.
Case Studies
Case Study 1: Transfection Efficiency of PDDA Nanoparticles
A comparative study evaluated the transfection efficiency of PDDA-based nanoparticles against conventional liposomal agents. Results indicated that while the overall transfection rates were lower than those achieved with liposomes at higher doses, the reduced cytotoxicity of PDDA nanoparticles allowed for safer applications in vivo .
Case Study 2: Hydrogel Formation and Drug Release
In another investigation, PDDA was incorporated into hydrogel matrices for drug delivery. The study found that hydrogels formed with PDDA exhibited favorable mechanical properties and sustained drug release profiles over several days, demonstrating its utility in therapeutic applications .
Advantages Over Traditional Monomers
The transition from petroleum-based monomers to bio-based alternatives like PDDA presents several advantages:
- Lower Toxicity : As highlighted in various studies, PDDA shows significantly lower cytotoxicity compared to its petroleum-derived counterparts.
- Sustainability : Sourced from renewable materials, PDDA contributes to reducing environmental impact.
- Versatility : Its properties allow for diverse applications ranging from coatings to biomedical uses.
Propiedades
IUPAC Name |
5-prop-2-enoyloxypentyl prop-2-enoate | |
---|---|---|
Source | PubChem | |
URL | https://pubchem.ncbi.nlm.nih.gov | |
Description | Data deposited in or computed by PubChem | |
InChI |
InChI=1S/C11H16O4/c1-3-10(12)14-8-6-5-7-9-15-11(13)4-2/h3-4H,1-2,5-9H2 | |
Source | PubChem | |
URL | https://pubchem.ncbi.nlm.nih.gov | |
Description | Data deposited in or computed by PubChem | |
InChI Key |
XAMCLRBWHRRBCN-UHFFFAOYSA-N | |
Source | PubChem | |
URL | https://pubchem.ncbi.nlm.nih.gov | |
Description | Data deposited in or computed by PubChem | |
Canonical SMILES |
C=CC(=O)OCCCCCOC(=O)C=C | |
Source | PubChem | |
URL | https://pubchem.ncbi.nlm.nih.gov | |
Description | Data deposited in or computed by PubChem | |
Molecular Formula |
C11H16O4 | |
Source | PubChem | |
URL | https://pubchem.ncbi.nlm.nih.gov | |
Description | Data deposited in or computed by PubChem | |
DSSTOX Substance ID |
DTXSID90190303 | |
Record name | 1,5-Pentanediyl diacrylate | |
Source | EPA DSSTox | |
URL | https://comptox.epa.gov/dashboard/DTXSID90190303 | |
Description | DSSTox provides a high quality public chemistry resource for supporting improved predictive toxicology. | |
Molecular Weight |
212.24 g/mol | |
Source | PubChem | |
URL | https://pubchem.ncbi.nlm.nih.gov | |
Description | Data deposited in or computed by PubChem | |
CAS No. |
36840-85-4 | |
Record name | 1,1′-(1,5-Pentanediyl) di-2-propenoate | |
Source | CAS Common Chemistry | |
URL | https://commonchemistry.cas.org/detail?cas_rn=36840-85-4 | |
Description | CAS Common Chemistry is an open community resource for accessing chemical information. Nearly 500,000 chemical substances from CAS REGISTRY cover areas of community interest, including common and frequently regulated chemicals, and those relevant to high school and undergraduate chemistry classes. This chemical information, curated by our expert scientists, is provided in alignment with our mission as a division of the American Chemical Society. | |
Explanation | The data from CAS Common Chemistry is provided under a CC-BY-NC 4.0 license, unless otherwise stated. | |
Record name | 1,5-Pentanediol diacrylate | |
Source | ChemIDplus | |
URL | https://pubchem.ncbi.nlm.nih.gov/substance/?source=chemidplus&sourceid=0036840854 | |
Description | ChemIDplus is a free, web search system that provides access to the structure and nomenclature authority files used for the identification of chemical substances cited in National Library of Medicine (NLM) databases, including the TOXNET system. | |
Record name | 1,5-Pentanediyl diacrylate | |
Source | EPA DSSTox | |
URL | https://comptox.epa.gov/dashboard/DTXSID90190303 | |
Description | DSSTox provides a high quality public chemistry resource for supporting improved predictive toxicology. | |
Record name | 1,5-pentanediyl diacrylate | |
Source | European Chemicals Agency (ECHA) | |
URL | https://echa.europa.eu/substance-information/-/substanceinfo/100.048.380 | |
Description | The European Chemicals Agency (ECHA) is an agency of the European Union which is the driving force among regulatory authorities in implementing the EU's groundbreaking chemicals legislation for the benefit of human health and the environment as well as for innovation and competitiveness. | |
Explanation | Use of the information, documents and data from the ECHA website is subject to the terms and conditions of this Legal Notice, and subject to other binding limitations provided for under applicable law, the information, documents and data made available on the ECHA website may be reproduced, distributed and/or used, totally or in part, for non-commercial purposes provided that ECHA is acknowledged as the source: "Source: European Chemicals Agency, http://echa.europa.eu/". Such acknowledgement must be included in each copy of the material. ECHA permits and encourages organisations and individuals to create links to the ECHA website under the following cumulative conditions: Links can only be made to webpages that provide a link to the Legal Notice page. | |
Record name | 1,5-PENTANEDIOL DIACRYLATE | |
Source | FDA Global Substance Registration System (GSRS) | |
URL | https://gsrs.ncats.nih.gov/ginas/app/beta/substances/7UA5KZC4S7 | |
Description | The FDA Global Substance Registration System (GSRS) enables the efficient and accurate exchange of information on what substances are in regulated products. Instead of relying on names, which vary across regulatory domains, countries, and regions, the GSRS knowledge base makes it possible for substances to be defined by standardized, scientific descriptions. | |
Explanation | Unless otherwise noted, the contents of the FDA website (www.fda.gov), both text and graphics, are not copyrighted. They are in the public domain and may be republished, reprinted and otherwise used freely by anyone without the need to obtain permission from FDA. Credit to the U.S. Food and Drug Administration as the source is appreciated but not required. | |
Retrosynthesis Analysis
AI-Powered Synthesis Planning: Our tool employs the Template_relevance Pistachio, Template_relevance Bkms_metabolic, Template_relevance Pistachio_ringbreaker, Template_relevance Reaxys, Template_relevance Reaxys_biocatalysis model, leveraging a vast database of chemical reactions to predict feasible synthetic routes.
One-Step Synthesis Focus: Specifically designed for one-step synthesis, it provides concise and direct routes for your target compounds, streamlining the synthesis process.
Accurate Predictions: Utilizing the extensive PISTACHIO, BKMS_METABOLIC, PISTACHIO_RINGBREAKER, REAXYS, REAXYS_BIOCATALYSIS database, our tool offers high-accuracy predictions, reflecting the latest in chemical research and data.
Strategy Settings
Precursor scoring | Relevance Heuristic |
---|---|
Min. plausibility | 0.01 |
Model | Template_relevance |
Template Set | Pistachio/Bkms_metabolic/Pistachio_ringbreaker/Reaxys/Reaxys_biocatalysis |
Top-N result to add to graph | 6 |
Feasible Synthetic Routes
Q1: Can 1,5-Pentanediol diacrylate cause allergic reactions?
A: While the provided research doesn't explicitly study allergic reactions, it does investigate the sensitizing potential of various diacrylates, including this compound, in guinea pigs. The study found that this compound exhibited an atypical sensitization pattern in the Freund's Complete Adjuvant Test (FCAT). [] While some animals showed sensitivity, the reactions were short-lived and diminished over time. This unusual pattern makes it difficult to definitively classify this compound as a sensitizer based on this study alone. Further research is needed to fully understand its potential to cause allergic reactions.
Descargo de responsabilidad e información sobre productos de investigación in vitro
Tenga en cuenta que todos los artículos e información de productos presentados en BenchChem están destinados únicamente con fines informativos. Los productos disponibles para la compra en BenchChem están diseñados específicamente para estudios in vitro, que se realizan fuera de organismos vivos. Los estudios in vitro, derivados del término latino "in vidrio", involucran experimentos realizados en entornos de laboratorio controlados utilizando células o tejidos. Es importante tener en cuenta que estos productos no se clasifican como medicamentos y no han recibido la aprobación de la FDA para la prevención, tratamiento o cura de ninguna condición médica, dolencia o enfermedad. Debemos enfatizar que cualquier forma de introducción corporal de estos productos en humanos o animales está estrictamente prohibida por ley. Es esencial adherirse a estas pautas para garantizar el cumplimiento de los estándares legales y éticos en la investigación y experimentación.