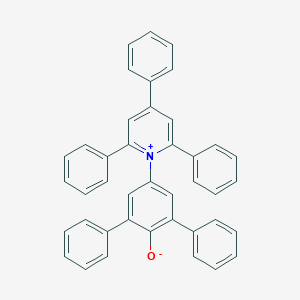
Tinte de Reichardt
Descripción general
Descripción
Synthesis Analysis
The synthesis of this compound is relatively complex and typically requires a chemical reaction. A common method involves reacting 2,6-diphenyl-4-methoxyphenol with 2,4,6-triphenylpyridine .Molecular Structure Analysis
The empirical formula of this compound is C41H29NO, and it has a molecular weight of 551.68 .Chemical Reactions Analysis
This compound can be used as a redox indicator to measure the reducing properties of substances. It is also a commonly used natural antioxidant that can be used to detect and evaluate the antioxidant properties of food, drugs, and cosmetics .Physical And Chemical Properties Analysis
This compound is a solid with a dark green to very dark grey color . It has a melting point of 271-275 °C (lit.) . It is slightly soluble in chloroform and ethyl acetate when sonicated .Aplicaciones Científicas De Investigación
Sonda de Polaridad del Solvente
El tinte de Reichardt se usa ampliamente como una sonda de polaridad del solvente en varias aplicaciones científicas . Juega un papel crucial en la determinación de los efectos del uso de diferentes solventes . Esto es particularmente importante en sistemas biológicos y en superficies .
Interacción de Cationes de Líquidos Iónicos
El tinte tiene una interacción específica con los cationes de los líquidos iónicos . En los casos en que la carga positiva del catión está deslocalizada en un anillo aromático como el imidazolio, los orbitales antienlazantes del sistema aromático cargado positivamente son muy similares en naturaleza y energía al LUMO del this compound . Esta interacción se puede usar para dilucidar la naturaleza de los cationes de los líquidos iónicos .
Solvatocromismo
El this compound es un tinte betaina solvatocrómico . El solvatocromismo es la dependencia de la longitud de onda de absorción de un tinte con respecto al solvente en el que está solvatado . Esta propiedad del this compound se ha utilizado para la determinación de la polaridad del solvente .
Solvatomorfismo
Un estudio sistemático de la influencia del solvente en el comportamiento de empaquetamiento cristalino del this compound demuestra que la estructura del ensamblaje formado en estado sólido depende de la naturaleza de las interacciones solvente-soluto presentes en la fase de solución
Mecanismo De Acción
Reichardt’s dye, also known as 2,6-Diphenyl-4-(2,4,6-triphenyl-1-pyridinio)phenolate, is an organic dye notable for its solvatochromic properties . This article will delve into the various aspects of its mechanism of action.
Target of Action
The primary target of Reichardt’s dye is the solvent in which it is dissolved. The dye interacts with the solvent molecules, leading to changes in its color .
Mode of Action
Reichardt’s dye exhibits solvatochromic behavior, meaning its color changes depending on the polarity of the solvent it is dissolved in . This is due to the differential solvation of the ground and excited states of the dye molecule . In negative solvatochromism, the ground state of the molecule is better stabilized by a more polar solvent, causing the energy required to reach the excited state to be larger compared to when dissolved in a non-polar solvent .
Biochemical Pathways
Its color-changing properties can be used to study the properties of various environments, including micellar environments and binary liquid mixtures .
Pharmacokinetics
The dye’s solubility in a wide range of solvents is a key factor influencing its behavior .
Result of Action
The primary result of Reichardt’s dye action is a change in color that varies across the entire visible spectrum depending on the polarity of the solvent . This provides a visual representation of the solvent’s polarity and can be used for various applications, including thermochromism, solvatochromism, piezochromism, and halochromism .
Action Environment
The action of Reichardt’s dye is highly dependent on the environmental factors, particularly the nature of the solvent in which it is dissolved . The dye’s color and solvatochromic behavior can be influenced by the solvent’s polarity . Furthermore, the dye’s stability can be affected by the presence of certain solvents .
Safety and Hazards
The compound is relatively safe under general use conditions . It is a non-flammable solid, but contact with flammable materials and high temperatures should be avoided . During operation, appropriate personal protective equipment, such as laboratory gloves and glasses, should be worn to avoid contact with skin and eyes .
Direcciones Futuras
Propiedades
IUPAC Name |
2,6-diphenyl-4-(2,4,6-triphenylpyridin-1-ium-1-yl)phenolate | |
---|---|---|
Source | PubChem | |
URL | https://pubchem.ncbi.nlm.nih.gov | |
Description | Data deposited in or computed by PubChem | |
InChI |
InChI=1S/C41H29NO/c43-41-37(31-18-8-2-9-19-31)28-36(29-38(41)32-20-10-3-11-21-32)42-39(33-22-12-4-13-23-33)26-35(30-16-6-1-7-17-30)27-40(42)34-24-14-5-15-25-34/h1-29H | |
Source | PubChem | |
URL | https://pubchem.ncbi.nlm.nih.gov | |
Description | Data deposited in or computed by PubChem | |
InChI Key |
UWOVWIIOKHRNKU-UHFFFAOYSA-N | |
Source | PubChem | |
URL | https://pubchem.ncbi.nlm.nih.gov | |
Description | Data deposited in or computed by PubChem | |
Canonical SMILES |
C1=CC=C(C=C1)C2=CC(=[N+](C(=C2)C3=CC=CC=C3)C4=CC(=C(C(=C4)C5=CC=CC=C5)[O-])C6=CC=CC=C6)C7=CC=CC=C7 | |
Source | PubChem | |
URL | https://pubchem.ncbi.nlm.nih.gov | |
Description | Data deposited in or computed by PubChem | |
Molecular Formula |
C41H29NO | |
Source | PubChem | |
URL | https://pubchem.ncbi.nlm.nih.gov | |
Description | Data deposited in or computed by PubChem | |
DSSTOX Substance ID |
DTXSID60373025 | |
Record name | Reichardt's dye | |
Source | EPA DSSTox | |
URL | https://comptox.epa.gov/dashboard/DTXSID60373025 | |
Description | DSSTox provides a high quality public chemistry resource for supporting improved predictive toxicology. | |
Molecular Weight |
551.7 g/mol | |
Source | PubChem | |
URL | https://pubchem.ncbi.nlm.nih.gov | |
Description | Data deposited in or computed by PubChem | |
CAS RN |
10081-39-7 | |
Record name | Betaine 30 | |
Source | CAS Common Chemistry | |
URL | https://commonchemistry.cas.org/detail?cas_rn=10081-39-7 | |
Description | CAS Common Chemistry is an open community resource for accessing chemical information. Nearly 500,000 chemical substances from CAS REGISTRY cover areas of community interest, including common and frequently regulated chemicals, and those relevant to high school and undergraduate chemistry classes. This chemical information, curated by our expert scientists, is provided in alignment with our mission as a division of the American Chemical Society. | |
Explanation | The data from CAS Common Chemistry is provided under a CC-BY-NC 4.0 license, unless otherwise stated. | |
Record name | 2,6-Diphenyl-4-(2,4,6-triphenyl-1-pyridinium)phenolate | |
Source | ChemIDplus | |
URL | https://pubchem.ncbi.nlm.nih.gov/substance/?source=chemidplus&sourceid=0010081397 | |
Description | ChemIDplus is a free, web search system that provides access to the structure and nomenclature authority files used for the identification of chemical substances cited in National Library of Medicine (NLM) databases, including the TOXNET system. | |
Record name | Reichardt's dye | |
Source | EPA DSSTox | |
URL | https://comptox.epa.gov/dashboard/DTXSID60373025 | |
Description | DSSTox provides a high quality public chemistry resource for supporting improved predictive toxicology. | |
Record name | 2,6-DIPHENYL-4-(2,4,6-TRIPHENYL-1-PYRIDINIUM)PHENOLATE | |
Source | FDA Global Substance Registration System (GSRS) | |
URL | https://gsrs.ncats.nih.gov/ginas/app/beta/substances/NN977V8PG5 | |
Description | The FDA Global Substance Registration System (GSRS) enables the efficient and accurate exchange of information on what substances are in regulated products. Instead of relying on names, which vary across regulatory domains, countries, and regions, the GSRS knowledge base makes it possible for substances to be defined by standardized, scientific descriptions. | |
Explanation | Unless otherwise noted, the contents of the FDA website (www.fda.gov), both text and graphics, are not copyrighted. They are in the public domain and may be republished, reprinted and otherwise used freely by anyone without the need to obtain permission from FDA. Credit to the U.S. Food and Drug Administration as the source is appreciated but not required. | |
Retrosynthesis Analysis
AI-Powered Synthesis Planning: Our tool employs the Template_relevance Pistachio, Template_relevance Bkms_metabolic, Template_relevance Pistachio_ringbreaker, Template_relevance Reaxys, Template_relevance Reaxys_biocatalysis model, leveraging a vast database of chemical reactions to predict feasible synthetic routes.
One-Step Synthesis Focus: Specifically designed for one-step synthesis, it provides concise and direct routes for your target compounds, streamlining the synthesis process.
Accurate Predictions: Utilizing the extensive PISTACHIO, BKMS_METABOLIC, PISTACHIO_RINGBREAKER, REAXYS, REAXYS_BIOCATALYSIS database, our tool offers high-accuracy predictions, reflecting the latest in chemical research and data.
Strategy Settings
Precursor scoring | Relevance Heuristic |
---|---|
Min. plausibility | 0.01 |
Model | Template_relevance |
Template Set | Pistachio/Bkms_metabolic/Pistachio_ringbreaker/Reaxys/Reaxys_biocatalysis |
Top-N result to add to graph | 6 |
Feasible Synthetic Routes
Descargo de responsabilidad e información sobre productos de investigación in vitro
Tenga en cuenta que todos los artículos e información de productos presentados en BenchChem están destinados únicamente con fines informativos. Los productos disponibles para la compra en BenchChem están diseñados específicamente para estudios in vitro, que se realizan fuera de organismos vivos. Los estudios in vitro, derivados del término latino "in vidrio", involucran experimentos realizados en entornos de laboratorio controlados utilizando células o tejidos. Es importante tener en cuenta que estos productos no se clasifican como medicamentos y no han recibido la aprobación de la FDA para la prevención, tratamiento o cura de ninguna condición médica, dolencia o enfermedad. Debemos enfatizar que cualquier forma de introducción corporal de estos productos en humanos o animales está estrictamente prohibida por ley. Es esencial adherirse a estas pautas para garantizar el cumplimiento de los estándares legales y éticos en la investigación y experimentación.