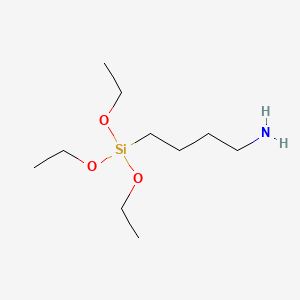
4-Aminobutyltriethoxysilane
Descripción general
Descripción
4-Aminobutyltriethoxysilane is an organosilane compound with the chemical formula C10H25NO3Si. It is commonly used as a coupling agent to improve the adhesion between organic and inorganic materials. This compound is particularly valuable in the production of epoxy nanocomposites, where it enhances the dielectric properties and thermal stability of the materials .
Aplicaciones Científicas De Investigación
4-Aminobutyltriethoxysilane has a wide range of applications in scientific research:
Biology: Employed in the functionalization of nanoparticles for targeted drug delivery systems.
Medicine: Utilized in the development of biocompatible coatings for medical implants.
Industry: Applied in the production of high-performance adhesives and sealants
Mecanismo De Acción
Target of Action
4-Aminobutyltriethoxysilane is primarily used as a chemical intermediate . It is known to act as a coupling agent, attaching easily with nanoparticle surfaces . This property makes it a valuable tool in the modification of nanoparticle surfaces, particularly in the creation of epoxy nanocomposites .
Mode of Action
The mode of action of this compound involves its interaction with nanoparticle surfaces. As a coupling agent, it forms bonds with these surfaces, thereby modifying their properties . This interaction results in changes to the nanoparticle surfaces, enhancing their compatibility with other substances and improving the overall performance of the resulting nanocomposites .
Biochemical Pathways
Its role as a coupling agent suggests that it may influence pathways related to surface adhesion and compatibility in nanoparticle-based systems .
Pharmacokinetics
It is known that the hydrolysis product of this compound is ethanol . Overexposure to ethanol, whether through skin absorption, inhalation, or ingestion, can have a narcotic effect .
Result of Action
The primary result of this compound’s action is the modification of nanoparticle surfaces. This modification enhances the performance of the resulting nanocomposites, making them suitable for high-performance electrical and thermal applications .
Action Environment
The action of this compound can be influenced by various environmental factors. For instance, its hydrolysis product, ethanol, can have varying effects depending on the conditions of exposure . Additionally, the efficacy and stability of this compound may be affected by factors such as temperature, pH, and the presence of other substances .
Análisis Bioquímico
Biochemical Properties
4-Aminobutyltriethoxysilane acts as a coupling agent and attaches easily with nanoparticle surfaces
Cellular Effects
The cellular effects of this compound are primarily observed in its role as a coupling agent in the synthesis of nanocomposites
Molecular Mechanism
It is known to attach easily with nanoparticle surfaces, suggesting potential binding interactions
Métodos De Preparación
Synthetic Routes and Reaction Conditions
4-Aminobutyltriethoxysilane can be synthesized from 3-cyanopropyltriethoxysilane through a two-step process. The first step involves the reduction of the nitrile group to an amine group, followed by the reaction with triethoxysilane .
Industrial Production Methods
In industrial settings, the production of this compound typically involves the use of large-scale reactors where the reaction conditions are carefully controlled to ensure high yield and purity. The process often includes purification steps such as distillation and crystallization to remove any impurities .
Análisis De Reacciones Químicas
Types of Reactions
4-Aminobutyltriethoxysilane undergoes various chemical reactions, including:
Hydrolysis: The ethoxy groups can be hydrolyzed to form silanol groups.
Condensation: The silanol groups can further condense to form siloxane bonds.
Substitution: The amine group can participate in nucleophilic substitution reactions
Common Reagents and Conditions
Hydrolysis: Typically carried out in the presence of water and a catalyst such as hydrochloric acid.
Condensation: Often facilitated by heating and the presence of a catalyst like acetic acid.
Substitution: Common reagents include alkyl halides and acyl chlorides
Major Products Formed
Hydrolysis: Produces silanol groups.
Condensation: Forms siloxane bonds, leading to polymeric structures.
Substitution: Results in the formation of various substituted amines
Comparación Con Compuestos Similares
Similar Compounds
- 3-Aminopropyltriethoxysilane
- N-(2-Aminoethyl)-3-aminopropyltrimethoxysilane
- (3-Glycidoxypropyl)trimethoxysilane
Uniqueness
4-Aminobutyltriethoxysilane is unique due to its longer alkyl chain, which provides greater flexibility and improved compatibility with a wider range of materials. This makes it particularly effective in applications requiring enhanced dielectric properties and thermal stability .
Propiedades
IUPAC Name |
4-triethoxysilylbutan-1-amine | |
---|---|---|
Source | PubChem | |
URL | https://pubchem.ncbi.nlm.nih.gov | |
Description | Data deposited in or computed by PubChem | |
InChI |
InChI=1S/C10H25NO3Si/c1-4-12-15(13-5-2,14-6-3)10-8-7-9-11/h4-11H2,1-3H3 | |
Source | PubChem | |
URL | https://pubchem.ncbi.nlm.nih.gov | |
Description | Data deposited in or computed by PubChem | |
InChI Key |
SWDDLRSGGCWDPH-UHFFFAOYSA-N | |
Source | PubChem | |
URL | https://pubchem.ncbi.nlm.nih.gov | |
Description | Data deposited in or computed by PubChem | |
Canonical SMILES |
CCO[Si](CCCCN)(OCC)OCC | |
Source | PubChem | |
URL | https://pubchem.ncbi.nlm.nih.gov | |
Description | Data deposited in or computed by PubChem | |
Molecular Formula |
C10H25NO3Si | |
Source | PubChem | |
URL | https://pubchem.ncbi.nlm.nih.gov | |
Description | Data deposited in or computed by PubChem | |
DSSTOX Substance ID |
DTXSID70184720 | |
Record name | 4-Aminobutyltriethoxysilane | |
Source | EPA DSSTox | |
URL | https://comptox.epa.gov/dashboard/DTXSID70184720 | |
Description | DSSTox provides a high quality public chemistry resource for supporting improved predictive toxicology. | |
Molecular Weight |
235.40 g/mol | |
Source | PubChem | |
URL | https://pubchem.ncbi.nlm.nih.gov | |
Description | Data deposited in or computed by PubChem | |
CAS No. |
3069-30-5 | |
Record name | 4-Aminobutyltriethoxysilane | |
Source | CAS Common Chemistry | |
URL | https://commonchemistry.cas.org/detail?cas_rn=3069-30-5 | |
Description | CAS Common Chemistry is an open community resource for accessing chemical information. Nearly 500,000 chemical substances from CAS REGISTRY cover areas of community interest, including common and frequently regulated chemicals, and those relevant to high school and undergraduate chemistry classes. This chemical information, curated by our expert scientists, is provided in alignment with our mission as a division of the American Chemical Society. | |
Explanation | The data from CAS Common Chemistry is provided under a CC-BY-NC 4.0 license, unless otherwise stated. | |
Record name | 4-Aminobutyltriethoxysilane | |
Source | ChemIDplus | |
URL | https://pubchem.ncbi.nlm.nih.gov/substance/?source=chemidplus&sourceid=0003069305 | |
Description | ChemIDplus is a free, web search system that provides access to the structure and nomenclature authority files used for the identification of chemical substances cited in National Library of Medicine (NLM) databases, including the TOXNET system. | |
Record name | 4-Aminobutyltriethoxysilane | |
Source | EPA DSSTox | |
URL | https://comptox.epa.gov/dashboard/DTXSID70184720 | |
Description | DSSTox provides a high quality public chemistry resource for supporting improved predictive toxicology. | |
Record name | 4-AMINOBUTYLTRIETHOXYSILANE | |
Source | FDA Global Substance Registration System (GSRS) | |
URL | https://gsrs.ncats.nih.gov/ginas/app/beta/substances/16M550E30K | |
Description | The FDA Global Substance Registration System (GSRS) enables the efficient and accurate exchange of information on what substances are in regulated products. Instead of relying on names, which vary across regulatory domains, countries, and regions, the GSRS knowledge base makes it possible for substances to be defined by standardized, scientific descriptions. | |
Explanation | Unless otherwise noted, the contents of the FDA website (www.fda.gov), both text and graphics, are not copyrighted. They are in the public domain and may be republished, reprinted and otherwise used freely by anyone without the need to obtain permission from FDA. Credit to the U.S. Food and Drug Administration as the source is appreciated but not required. | |
Retrosynthesis Analysis
AI-Powered Synthesis Planning: Our tool employs the Template_relevance Pistachio, Template_relevance Bkms_metabolic, Template_relevance Pistachio_ringbreaker, Template_relevance Reaxys, Template_relevance Reaxys_biocatalysis model, leveraging a vast database of chemical reactions to predict feasible synthetic routes.
One-Step Synthesis Focus: Specifically designed for one-step synthesis, it provides concise and direct routes for your target compounds, streamlining the synthesis process.
Accurate Predictions: Utilizing the extensive PISTACHIO, BKMS_METABOLIC, PISTACHIO_RINGBREAKER, REAXYS, REAXYS_BIOCATALYSIS database, our tool offers high-accuracy predictions, reflecting the latest in chemical research and data.
Strategy Settings
Precursor scoring | Relevance Heuristic |
---|---|
Min. plausibility | 0.01 |
Model | Template_relevance |
Template Set | Pistachio/Bkms_metabolic/Pistachio_ringbreaker/Reaxys/Reaxys_biocatalysis |
Top-N result to add to graph | 6 |
Feasible Synthetic Routes
Q1: How does 4-Aminobutyltriethoxysilane (ABTES) enhance the properties of epoxy resins?
A1: ABTES acts as a coupling agent, enhancing the compatibility between inorganic fillers and the organic epoxy matrix. This is achieved through its bifunctional structure:
- Silane group (Si-OEt): This group hydrolyzes and condenses with hydroxyl groups present on the surface of inorganic fillers like TiO2 [] or zirconia-silica [].
- Amine group (NH2): This group reacts with the epoxy groups in the resin during the curing process [, ].
Q2: How does the concentration of ABTES-modified TiO2 nanoparticles affect the antimicrobial properties of epoxy nanocomposites?
A: Research indicates that increasing the concentration of this compound-modified TiO2 nanoparticles (TiO2-ABTES) in epoxy nanocomposites leads to enhanced antimicrobial activity. This was demonstrated in a study where TiO2-ABTES nanoparticles were incorporated into a diglycidyl ethers of bisphenol-A (DGEBA) matrix at varying concentrations []. The results showed that the nanocomposite film with 7 wt% TiO2-ABTES exhibited the largest inhibition zone against tested pathogens, suggesting a concentration-dependent antimicrobial effect [].
Q3: Can this compound (ABTES) negatively impact the properties of the materials it's incorporated into?
A: While ABTES generally improves material properties, its effectiveness depends on factors like concentration and the specific components involved. For instance, in a study using ABTES to modify zirconia-silica (ZS) fillers in a urethane dimethacrylate (UDMA) matrix, high concentrations of ABTES led to the formation of a separate interphase []. This negatively impacted compatibility, suggesting that an optimal ABTES concentration is crucial for achieving desired material properties.
Q4: What analytical techniques are used to study the effects of ABTES modification on material properties?
A4: Researchers utilize a variety of techniques to characterize ABTES-modified materials. Some common methods include:
- Fourier Transform Infrared Spectroscopy (FTIR): Used to identify functional groups and confirm successful silane modification of nanoparticles and their interaction with the matrix [, ].
- Scanning Electron Microscopy (SEM) and Atomic Force Microscopy (AFM): Provide visual information about the dispersion of nanoparticles within the matrix and surface morphology [].
- Dielectric Spectroscopy: Helps analyze molecular mobility and interactions within the material, particularly how the presence of fillers and silane treatments affect polymer chain dynamics [].
Descargo de responsabilidad e información sobre productos de investigación in vitro
Tenga en cuenta que todos los artículos e información de productos presentados en BenchChem están destinados únicamente con fines informativos. Los productos disponibles para la compra en BenchChem están diseñados específicamente para estudios in vitro, que se realizan fuera de organismos vivos. Los estudios in vitro, derivados del término latino "in vidrio", involucran experimentos realizados en entornos de laboratorio controlados utilizando células o tejidos. Es importante tener en cuenta que estos productos no se clasifican como medicamentos y no han recibido la aprobación de la FDA para la prevención, tratamiento o cura de ninguna condición médica, dolencia o enfermedad. Debemos enfatizar que cualquier forma de introducción corporal de estos productos en humanos o animales está estrictamente prohibida por ley. Es esencial adherirse a estas pautas para garantizar el cumplimiento de los estándares legales y éticos en la investigación y experimentación.