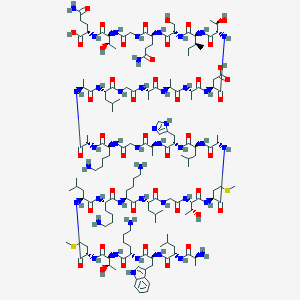
Dermaseptin
Descripción general
Descripción
Dermaseptins are a family of antimicrobial peptides isolated from the skin secretions of Hylid frogs, particularly from the Agalychnis and Phyllomedusa families . These peptides are known for their broad-spectrum antimicrobial activity against bacteria, parasites, protozoa, and viruses . Dermaseptins are characterized by their α-helical structure and polycationic nature, which contribute to their ability to interact with microbial membranes .
Métodos De Preparación
Synthetic Routes and Reaction Conditions: Dermaseptins can be synthesized chemically using solid-phase peptide synthesis (SPPS). This method involves the stepwise addition of amino acids to a growing peptide chain anchored to a solid resin. The process includes deprotection and coupling steps, typically using reagents such as N,N’-diisopropylcarbodiimide (DIC) and N-hydroxybenzotriazole (HOBt) for coupling, and trifluoroacetic acid (TFA) for deprotection .
Industrial Production Methods: Industrial production of dermaseptins involves recombinant DNA technology. The genes encoding dermaseptins are cloned into suitable expression vectors and introduced into host cells, such as Escherichia coli or yeast. The expressed peptides are then purified using chromatographic techniques, including reverse-phase high-performance liquid chromatography (RP-HPLC) .
Análisis De Reacciones Químicas
Key Chemical Reactions and Interactions
-
Interaction with Cell Membranes: Dermaseptins, being cationic AMPs, interact with the anionic phospholipid composition of cell membranes through electrostatic attraction . This interaction is crucial for their antimicrobial and antitumor activity.
-
Membrane Disruption: Dermaseptins disrupt microbial membranes through mechanisms such as the "barrel-stave" model and the "carpet-like" mechanism .
-
Alpha-Helical Conformation: Dermaseptins adopt an alpha-helical structure in membrane-mimicking environments, which facilitates their insertion into cell membranes . The percentage of α-helicity can be determined using circular dichroism (CD) spectroscopy . For instance, this compound-PP exhibits a typical α-helical structure in 50% TFE solution, with approximately 88% α-helicity .
-
Effects on Cancer Cells: this compound-PP induces apoptosis in cancer cells via both endogenous mitochondrial and exogenous death receptor pathways . This involves the increased expression of proteins such as cytochrome c, Apaf-1, caspase-9, caspase-3, Fas, FasL, and FADD .
Impact of Structural Modifications on Activity
Structural modifications, such as amino acid substitutions or deletions, can alter the activity and toxicity of dermaseptins .
-
Example: The this compound derivative K4S4(1-16) was created by substituting methionine at position 4 with lysine and deleting the 12 C-terminal residues of this compound S4 .
Data Tables
From Evaluation of the Antibacterial Activity of New this compound
Peptides | Sequence * | Parameters ** |
---|---|---|
Length | ||
S4 (Native) | ALWMTLLKKVLKAAAKAALNAVLVGANA | 28 |
K4K20S4 | ALWKTLLKKVLKAAAKAALKAVLVGANA | 28 |
K4S4(1-16) | ALWKTLLKKVLKAAAK | 16 |
B2 (Native) | GLWSKIKEVGKEAAKAAAKAAGKAALGAVSEAV | 33 |
K3K4B2 | GLKKKIKEVGKEAAKAAAKAAGKAALGAVSEAV | 33 |
*One-letter code for the amino-acid sequence of these peptides, taking as reference the sequence and length of native peptides DRS-S4 and DRS-B2
**MW: Molecular Weight; H: Hydrophobicity; μH: Hydrophobic Moment.
From Evaluation of the Antibacterial Activity of New this compound
Peptides | CC50 Hep-2 Cells (μg/mL) | A. baumannii MIC (μg/mL) | A. baumannii MBC (μg/mL) |
---|---|---|---|
S4 | 16.51 | 12.5 | 25 |
K4S4(1-16) | 68.9 | 6.25 | 12.5 |
K4K20S4 | 75.71 | 3.125 | 6.25 |
B2 | 30.4 | 12.5 | 25 |
K3K4B2 | 61.25 | 6.25 | 12.5 |
meropenem | ND | 32 | 64 |
CC50: peptide concentration that causes 50% cytotoxicity in HEp-2 cells for this compound S4 and derivatives (μg/mL); ND, not determined; MIC: Minimum Inhibitory Concentration (μg/mL); MBC: Minimal Bactericidal Concentration (μg/mL).
Aplicaciones Científicas De Investigación
Antimicrobial Properties
Broad-Spectrum Antimicrobial Activity
Dermaseptins have demonstrated potent antimicrobial effects against various pathogens, including bacteria, fungi, and viruses. They act primarily by disrupting microbial membranes, leading to cell lysis. Key findings include:
- Effectiveness Against Bacteria : Dermaseptin exhibits strong activity against both Gram-positive and Gram-negative bacteria, with minimum inhibitory concentrations (MICs) often reported as low as 0.1 mg/mL for strains like Escherichia coli and Staphylococcus aureus .
- Resistance to Multidrug-Resistant Strains : Studies have shown that certain derivatives of this compound maintain efficacy against multidrug-resistant bacterial strains, making them promising candidates for developing new antibiotics .
Anticancer Applications
Selective Cytotoxicity Towards Cancer Cells
Research has indicated that Dermaseptins possess selective cytotoxicity against various cancer cell lines while exhibiting lower toxicity to normal cells. Notable studies include:
- This compound-PH : This peptide was found to inhibit proliferation in several cancer cell lines, such as MCF-7 (breast cancer) and H157 (lung cancer), with IC50 values ranging from 0.69 μM to 11.8 μM .
- In Vivo Anti-Tumor Activity : In animal models, this compound-PP showed significant anti-tumor effects in H157 subcutaneous tumor models without causing severe side effects . The mechanisms involved include apoptosis induction and membrane disruption of cancer cells.
Safety and Toxicity
Safety assessments have shown that while Dermaseptins are effective against pathogens and cancer cells, they generally exhibit low hemolytic activity towards red blood cells, indicating a favorable safety profile for potential therapeutic applications .
Future Research Directions
Despite the promising results obtained so far, further research is needed to explore the full therapeutic potential of Dermaseptins:
- Clinical Trials : There is a lack of clinical trials assessing the efficacy and safety of Dermaseptins in humans. Future studies should focus on this aspect to validate their use in clinical settings.
- Mechanistic Studies : More detailed investigations into the mechanisms of action will help optimize these peptides for therapeutic use.
Summary Table of Key Findings
Application Area | Key Findings |
---|---|
Antimicrobial | Effective against Gram-positive/negative bacteria; low MICs reported |
Anticancer | Selective cytotoxicity; effective against various cancer cell lines |
Mechanism | Membrane disruption; apoptosis induction |
Safety Profile | Low hemolytic activity; generally safe for normal cells |
Mecanismo De Acción
Dermaseptins exert their effects by interacting with microbial membranes. Their polycationic nature allows them to bind to the negatively charged components of microbial membranes. Upon binding, they insert into the membrane and adopt an α-helical structure, forming pores that disrupt membrane integrity. This leads to leakage of cellular contents and cell death . In cancer cells, dermaseptins can induce apoptosis through interactions with cell surface receptors and intracellular targets .
Comparación Con Compuestos Similares
Dermaseptins are unique due to their broad-spectrum antimicrobial activity and low cytotoxicity to mammalian cells. Similar compounds include:
Magainins: Antimicrobial peptides from the skin of the African clawed frog, Xenopus laevis.
Defensins: A family of antimicrobial peptides found in plants, insects, and mammals.
Dermaseptins are distinguished by their higher potency and broader spectrum of activity compared to these similar compounds .
Actividad Biológica
Dermaseptins are a class of antimicrobial peptides (AMPs) primarily derived from the skin secretions of Phyllomedusa frogs. These peptides exhibit a broad spectrum of biological activities, including antimicrobial, antiviral, and antitumor effects. This article explores the biological activity of dermaseptins, focusing on their antimicrobial properties, mechanisms of action, and potential therapeutic applications.
Overview of Dermaseptins
Dermaseptins are characterized by their α-helical structure and cationic nature , which contribute to their ability to interact with microbial membranes. They are known for their efficacy against various pathogens, including bacteria, fungi, parasites, and viruses. The primary dermaseptins studied include dermaseptin S1, S4, S9, and B2, each exhibiting unique properties and activities.
Dermaseptins exert their antimicrobial effects primarily through membrane disruption. They bind to the anionic phospholipids present in microbial membranes, leading to increased permeability and ultimately cell lysis. The binding affinity varies among different dermaseptins, influencing their effectiveness against specific pathogens.
Efficacy Against Bacterial Strains
Numerous studies have documented the antimicrobial efficacy of dermaseptins against both Gram-positive and Gram-negative bacteria. The minimum inhibitory concentrations (MICs) for various dermaseptins are summarized in Table 1.
This compound | Target Pathogen | MIC (µg/mL) |
---|---|---|
This compound S4 | Staphylococcus aureus | 12.5 |
This compound B2 | Escherichia coli | 6.25 |
This compound K4 | Pseudomonas aeruginosa | 3.125 |
This compound S9 | Acinetobacter baumannii | 1.8 |
Case Studies
-
Antimicrobial Activity Against Drug-Resistant Strains :
A study evaluated the activity of this compound-SS1 against drug-resistant E. coli. Results indicated significant antimicrobial activity, with a therapeutic index favoring its use over conventional antibiotics . -
In Vitro Studies on Viral Infections :
Research on analogs of this compound B2 demonstrated their potential as antiviral agents against the Zika virus. The peptides inhibited viral entry into host cells without exhibiting cytotoxicity at effective concentrations . -
Antitumor Effects :
This compound B2 has shown antiproliferative effects on various tumor cell lines, suggesting a dual role in antimicrobial and antitumor activity .
Toxicity and Selectivity
While dermaseptins are potent antimicrobials, their cytotoxicity towards human cells is a critical consideration for therapeutic use. Studies report that certain derivatives exhibit lower cytotoxicity compared to conventional antibiotics while maintaining antimicrobial efficacy . For example, the derivative K4S4(1-16) displayed a CC50 (cytotoxic concentration for 50% of cells) greater than 61.25 μg/mL, indicating a favorable safety profile .
Future Directions
The potential applications of dermaseptins in medicine are vast but require further research to elucidate their mechanisms fully and optimize their structures for enhanced efficacy and safety. Future studies should focus on:
- In Vivo Efficacy : Evaluating the effectiveness of dermaseptins in animal models to confirm their therapeutic potential.
- Clinical Trials : Conducting clinical trials to assess safety and efficacy in humans.
- Structural Modifications : Investigating how modifications to peptide sequences can improve selectivity and reduce toxicity.
Q & A
Basic Research Questions
Q. What experimental methods are used to analyze the membrane-binding mechanisms of dermaseptin analogues?
Surface plasmon resonance (SPR) is a key method to study interactions between this compound derivatives and model lipid bilayers. This technique distinguishes between superficial adhesion and deeper insertion into membranes by calculating apparent affinity constants for each binding stage. For example, a two-stage mechanism was identified for this compound S4 derivatives, where cytolytic activity correlated more strongly with insertion affinity than initial adhesion . SPR data can be validated using proteolytic cleavage assays to confirm membrane penetration (e.g., K4K20-S4 showed protease resistance when inserted into bilayers) .
Q. How is the cytotoxicity of this compound derivatives evaluated in vitro?
Cytotoxicity is typically assessed using hemolysis assays (e.g., measuring hemoglobin release from red blood cells) and cell viability tests like MTT assays. For instance, this compound S4 derivatives with increased positive charge (e.g., K4K20-S4) showed reduced hemolytic activity while retaining antimicrobial potency, indicating selectivity for microbial membranes . Dose-response curves are critical to determine 50% cytotoxic concentrations (CC₅₀) and compare toxicity across cell types (e.g., mammalian vs. insect cells) .
Q. What structural features of this compound are critical for antimicrobial activity?
Dermaseptins are amphipathic α-helical peptides with cationic N-terminal domains and hydrophobic C-terminal regions. The cationic residues facilitate electrostatic interactions with negatively charged microbial membranes, while hydrophobicity enables membrane penetration. Truncation studies show that the N-terminal 16 residues of this compound S4 retain activity, but further shortening reduces potency, highlighting the importance of peptide length . Modifications like lysine substitutions enhance charge and selectivity .
Advanced Research Questions
Q. How can researchers resolve contradictions in the relationship between peptide length and antimicrobial efficacy?
While longer dermaseptins (28–34 residues) generally show higher activity, truncated analogues (e.g., 13-mer DP-1) can retain potency if designed with optimized charge-to-length ratios. For example, C-terminal truncations combined with N-terminal charge enhancements (e.g., TAT fusion in DP-2) improve cell penetration without compromising selectivity . Comparative studies using circular dichroism (CD) and NMR can reveal structural retention in shorter peptides .
Q. What methodologies are used to assess the antiviral activity of this compound derivatives?
Antiviral efficacy is tested using plaque reduction assays, viral titer quantification, and MTT-based cell viability in infected cultures. For Dengue virus type 2, this compound 01 exhibited a 50% effective concentration (EC₅₀) of 15 µg/mL in C6/36 cells, with parallel cytotoxicity assessments to confirm selectivity (CC₅₀ > 1000 µg/mL in mammalian cells) . Computational docking (e.g., against SARS-CoV-2 spike protein) and in silico affinity studies are also employed to predict antiviral potential .
Q. How do researchers design this compound analogues to balance antimicrobial potency and low cytotoxicity?
Key strategies include:
- Charge modulation : Increasing positive charge (e.g., lysine substitutions) enhances microbial membrane targeting but must be balanced to avoid nonspecific mammalian cell lysis .
- Hydrophobicity adjustment : Reducing hydrophobicity indices (e.g., via residue substitutions) lowers hemolytic activity while preserving antimicrobial effects .
- Structural stabilization : C-terminal amidation or α-helix stabilization (via NMR-guided design) improves membrane insertion efficiency .
Q. What experimental approaches elucidate the role of this compound in immune modulation?
this compound S1 stimulates polymorphonuclear leukocytes (PMNs) by enhancing reactive oxygen species (ROS) production and exocytosis via protein kinase C (PKC) and calcium signaling pathways. Methodologies include:
- Flow cytometry to measure ROS bursts.
- Myeloperoxidase release assays.
- Pharmacological inhibitors (e.g., GF 109203X for PKC) to dissect signaling pathways .
Q. Methodological Considerations
Q. How should researchers analyze conflicting data on membrane-binding models (e.g., one-stage vs. two-stage binding)?
SPR-derived binding isotherms should be fit to multiple interaction models (e.g., Langmuir vs. two-stage mechanisms) and validated with complementary techniques like fluorescence quenching or proteolytic protection assays. For this compound S4, SPR data aligned with a two-stage model where insertion affinity, not initial adhesion, dictated cytotoxicity . Energy transfer experiments (e.g., FRET) can further quantify peptide aggregation states on membranes .
Q. What statistical and computational tools are recommended for SAR studies of this compound?
- Molecular dynamics simulations : To predict membrane interactions and helical stability .
- Quantitative structure-activity relationship (QSAR) models : Correlate physicochemical properties (charge, hydrophobicity) with MIC/CC₅₀ values .
- NMR spectroscopy : Resolve 3D structures of derivatives to identify critical motifs (e.g., amphipathic helices) .
Q. How can researchers address discrepancies in cytotoxicity across cell types?
Cross-cell-type comparisons (e.g., erythrocytes vs. epithelial cells) and barrier complexity assessments (e.g., mucus layers) are essential. For example, this compound S4 derivatives showed reduced toxicity in human RBCs compared to bacterial cells due to differences in membrane composition . Use confocal microscopy to visualize peptide localization and membrane disruption in real time .
Propiedades
IUPAC Name |
(2S)-5-amino-2-[[(2S,3R)-2-[[2-[[(2S)-5-amino-2-[[(2S)-2-[[(2S,3S)-2-[[(2S,3R)-2-[[(2S)-2-[[(2S)-2-[[(2S)-2-[[(2S)-2-[[2-[[(2S)-2-[[(2S)-2-[[(2S)-2-[[(2S)-6-amino-2-[[2-[[(2S)-2-[[(2S)-2-[[(2S)-2-[[(2S)-2-[[(2S)-2-[[(2S,3R)-2-[[2-[[(2S)-2-[[(2S)-6-amino-2-[[(2S)-6-amino-2-[[(2S)-2-[[(2S)-2-[[(2S,3R)-2-[[(2S)-6-amino-2-[[(2S)-2-[[(2S)-2-[[(2S)-2-aminopropanoyl]amino]-4-methylpentanoyl]amino]-3-(1H-indol-3-yl)propanoyl]amino]hexanoyl]amino]-3-hydroxybutanoyl]amino]-4-methylsulfanylbutanoyl]amino]-4-methylpentanoyl]amino]hexanoyl]amino]hexanoyl]amino]-4-methylpentanoyl]amino]acetyl]amino]-3-hydroxybutanoyl]amino]-4-methylsulfanylbutanoyl]amino]propanoyl]amino]-4-methylpentanoyl]amino]-3-(1H-imidazol-5-yl)propanoyl]amino]propanoyl]amino]acetyl]amino]hexanoyl]amino]propanoyl]amino]propanoyl]amino]-4-methylpentanoyl]amino]acetyl]amino]propanoyl]amino]propanoyl]amino]propanoyl]amino]-3-carboxypropanoyl]amino]-3-hydroxybutanoyl]amino]-3-methylpentanoyl]amino]-3-hydroxypropanoyl]amino]-5-oxopentanoyl]amino]acetyl]amino]-3-hydroxybutanoyl]amino]-5-oxopentanoic acid | |
---|---|---|
Source | PubChem | |
URL | https://pubchem.ncbi.nlm.nih.gov | |
Description | Data deposited in or computed by PubChem | |
InChI |
InChI=1S/C152H257N43O44S2/c1-27-77(12)118(147(233)190-110(70-196)146(232)178-98(44-46-111(158)201)131(217)163-68-115(205)192-120(87(22)198)149(235)181-101(152(238)239)45-47-112(159)202)193-151(237)122(89(24)200)195-145(231)109(63-117(207)208)185-129(215)84(19)169-126(212)81(16)168-125(211)80(15)167-113(203)66-164-132(218)102(56-72(2)3)183-128(214)83(18)170-127(213)82(17)172-134(220)94(40-30-34-50-153)174-114(204)67-162-124(210)79(14)171-140(226)108(62-91-65-160-71-166-91)189-143(229)105(59-75(8)9)184-130(216)85(20)173-135(221)99(48-54-240-25)179-148(234)119(86(21)197)191-116(206)69-165-133(219)103(57-73(4)5)186-137(223)96(42-32-36-52-155)175-136(222)95(41-31-35-51-154)176-141(227)106(60-76(10)11)187-138(224)100(49-55-241-26)180-150(236)121(88(23)199)194-139(225)97(43-33-37-53-156)177-144(230)107(61-90-64-161-93-39-29-28-38-92(90)93)188-142(228)104(58-74(6)7)182-123(209)78(13)157/h28-29,38-39,64-65,71-89,94-110,118-122,161,196-200H,27,30-37,40-63,66-70,153-157H2,1-26H3,(H2,158,201)(H2,159,202)(H,160,166)(H,162,210)(H,163,217)(H,164,218)(H,165,219)(H,167,203)(H,168,211)(H,169,212)(H,170,213)(H,171,226)(H,172,220)(H,173,221)(H,174,204)(H,175,222)(H,176,227)(H,177,230)(H,178,232)(H,179,234)(H,180,236)(H,181,235)(H,182,209)(H,183,214)(H,184,216)(H,185,215)(H,186,223)(H,187,224)(H,188,228)(H,189,229)(H,190,233)(H,191,206)(H,192,205)(H,193,237)(H,194,225)(H,195,231)(H,207,208)(H,238,239)/t77-,78-,79-,80-,81-,82-,83-,84-,85-,86+,87+,88+,89+,94-,95-,96-,97-,98-,99-,100-,101-,102-,103-,104-,105-,106-,107-,108-,109-,110-,118-,119-,120-,121-,122-/m0/s1 | |
Source | PubChem | |
URL | https://pubchem.ncbi.nlm.nih.gov | |
Description | Data deposited in or computed by PubChem | |
InChI Key |
YFHLIDBAPTWLGU-CTKMSOPVSA-N | |
Source | PubChem | |
URL | https://pubchem.ncbi.nlm.nih.gov | |
Description | Data deposited in or computed by PubChem | |
Canonical SMILES |
CCC(C)C(C(=O)NC(CO)C(=O)NC(CCC(=O)N)C(=O)NCC(=O)NC(C(C)O)C(=O)NC(CCC(=O)N)C(=O)O)NC(=O)C(C(C)O)NC(=O)C(CC(=O)O)NC(=O)C(C)NC(=O)C(C)NC(=O)C(C)NC(=O)CNC(=O)C(CC(C)C)NC(=O)C(C)NC(=O)C(C)NC(=O)C(CCCCN)NC(=O)CNC(=O)C(C)NC(=O)C(CC1=CN=CN1)NC(=O)C(CC(C)C)NC(=O)C(C)NC(=O)C(CCSC)NC(=O)C(C(C)O)NC(=O)CNC(=O)C(CC(C)C)NC(=O)C(CCCCN)NC(=O)C(CCCCN)NC(=O)C(CC(C)C)NC(=O)C(CCSC)NC(=O)C(C(C)O)NC(=O)C(CCCCN)NC(=O)C(CC2=CNC3=CC=CC=C32)NC(=O)C(CC(C)C)NC(=O)C(C)N | |
Source | PubChem | |
URL | https://pubchem.ncbi.nlm.nih.gov | |
Description | Data deposited in or computed by PubChem | |
Isomeric SMILES |
CC[C@H](C)[C@@H](C(=O)N[C@@H](CO)C(=O)N[C@@H](CCC(=O)N)C(=O)NCC(=O)N[C@@H]([C@@H](C)O)C(=O)N[C@@H](CCC(=O)N)C(=O)O)NC(=O)[C@H]([C@@H](C)O)NC(=O)[C@H](CC(=O)O)NC(=O)[C@H](C)NC(=O)[C@H](C)NC(=O)[C@H](C)NC(=O)CNC(=O)[C@H](CC(C)C)NC(=O)[C@H](C)NC(=O)[C@H](C)NC(=O)[C@H](CCCCN)NC(=O)CNC(=O)[C@H](C)NC(=O)[C@H](CC1=CN=CN1)NC(=O)[C@H](CC(C)C)NC(=O)[C@H](C)NC(=O)[C@H](CCSC)NC(=O)[C@H]([C@@H](C)O)NC(=O)CNC(=O)[C@H](CC(C)C)NC(=O)[C@H](CCCCN)NC(=O)[C@H](CCCCN)NC(=O)[C@H](CC(C)C)NC(=O)[C@H](CCSC)NC(=O)[C@H]([C@@H](C)O)NC(=O)[C@H](CCCCN)NC(=O)[C@H](CC2=CNC3=CC=CC=C32)NC(=O)[C@H](CC(C)C)NC(=O)[C@H](C)N | |
Source | PubChem | |
URL | https://pubchem.ncbi.nlm.nih.gov | |
Description | Data deposited in or computed by PubChem | |
Molecular Formula |
C152H257N43O44S2 | |
Source | PubChem | |
URL | https://pubchem.ncbi.nlm.nih.gov | |
Description | Data deposited in or computed by PubChem | |
Molecular Weight |
3455.1 g/mol | |
Source | PubChem | |
URL | https://pubchem.ncbi.nlm.nih.gov | |
Description | Data deposited in or computed by PubChem | |
CAS No. |
136212-91-4 | |
Record name | Dermaseptin | |
Source | ChemIDplus | |
URL | https://pubchem.ncbi.nlm.nih.gov/substance/?source=chemidplus&sourceid=0136212914 | |
Description | ChemIDplus is a free, web search system that provides access to the structure and nomenclature authority files used for the identification of chemical substances cited in National Library of Medicine (NLM) databases, including the TOXNET system. | |
Descargo de responsabilidad e información sobre productos de investigación in vitro
Tenga en cuenta que todos los artículos e información de productos presentados en BenchChem están destinados únicamente con fines informativos. Los productos disponibles para la compra en BenchChem están diseñados específicamente para estudios in vitro, que se realizan fuera de organismos vivos. Los estudios in vitro, derivados del término latino "in vidrio", involucran experimentos realizados en entornos de laboratorio controlados utilizando células o tejidos. Es importante tener en cuenta que estos productos no se clasifican como medicamentos y no han recibido la aprobación de la FDA para la prevención, tratamiento o cura de ninguna condición médica, dolencia o enfermedad. Debemos enfatizar que cualquier forma de introducción corporal de estos productos en humanos o animales está estrictamente prohibida por ley. Es esencial adherirse a estas pautas para garantizar el cumplimiento de los estándares legales y éticos en la investigación y experimentación.