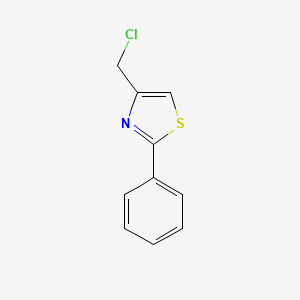
4-(Chloromethyl)-2-phenyl-1,3-thiazole
Descripción general
Descripción
4-(Chloromethyl)-2-phenyl-1,3-thiazole (CAS: 4771-31-7) is a substituted thiazole derivative characterized by a chloromethyl (-CH2Cl) group at the 4-position and a phenyl group at the 2-position of the thiazole ring. Its molecular formula is C10H8ClNS, with a molecular weight of 209.69 g/mol . This compound is structurally significant due to its reactive chloromethyl group, which facilitates further functionalization in synthetic chemistry, particularly in the development of antimicrobial agents, enzyme inhibitors, and hybrid pharmacophores . Its synthesis typically involves multistep reactions, such as coupling 4-(chloromethyl)benzoyl chloride with aminothiazoles under controlled conditions .
Métodos De Preparación
General Synthetic Strategies
The preparation of 4-(Chloromethyl)-2-phenyl-1,3-thiazole typically involves constructing the thiazole ring followed by chloromethylation or employing chloromethylated precursors in cyclization reactions. The synthetic approaches can be broadly categorized as:
- Cyclization of appropriate precursors containing phenyl and chloromethyl functionalities
- Chloromethylation of preformed 2-phenylthiazole derivatives
- One-pot reactions involving allyl isothiocyanates and chlorinating agents
Specific Preparation Methods
Preparation of this compound via Chlorination of Allyl Isothiocyanate Derivatives (Patent Method)
A more general and industrially relevant method for preparing chloromethyl-substituted thiazoles involves the reaction of allyl isothiocyanate with chlorinating agents under controlled temperature and solvent conditions. This process is described in detail for the analogous compound 2-chloro-5-chloromethyl-1,3-thiazole but is adaptable to this compound with appropriate substituents.
Step a): Chlorination Reaction
- React allyl isothiocyanate (or substituted derivatives) with 1 to 2 mol equivalents of chlorinating agent per mol of allyl isothiocyanate.
- Chlorinating agents include chlorine gas, sulfuryl chloride, phosphorus pentachloride, phosphorus trichloride, and phosphorus oxychloride.
- Reaction temperature: −40°C to +30°C (preferably −20°C to 0°C).
- Solvent: Inert solvents such as benzene, toluene, halogenated hydrocarbons (e.g., dichloromethane, 1,2-dichloroethane), ethers (e.g., tetrahydrofuran), nitriles (e.g., acetonitrile), or amides (e.g., dimethylformamide).
- Stirring time: 5 minutes to 5 hours (preferably 20 minutes to 2 hours).
Step b): Oxidation and Halogenation
- Add 1 to 5 mol equivalents of an oxidizing agent per mol of allyl isothiocyanate to the reaction mixture.
- Oxidizing agents include peroxy acids (peracetic acid, m-chloroperbenzoic acid), hydrogen peroxide mixtures, organic peroxides, quinones, or halogenating agents such as N-chloro- or N-bromo-succinimide.
- Reaction temperature: 0°C to boiling point of solvent (preferably 20°C to 80°C).
- Initiators like dibenzoyl peroxide or azobisisobutyronitrile may be used to accelerate halogenation.
-
- Precipitated imides (e.g., succinimide) are filtered off.
- The reaction mixture is washed with basic solutions (e.g., sodium bicarbonate or carbonate) to neutralize acids.
- The solvent is removed by distillation.
- The crude product is purified by distillation under reduced pressure and crystallized from aliphatic hydrocarbons (e.g., hexane, heptane) or ethers/esters.
- Crystallization temperatures range from 0°C to −40°C.
This process is advantageous for its one-pot nature, high purity, and yield of the chloromethyl thiazole compound.
Comparative Data Table of Preparation Methods
Research Findings and Notes
The chlorination method using allyl isothiocyanate and chlorinating agents is well-documented for producing chloromethyl-substituted thiazoles with high purity and yield. It is industrially scalable and allows for one-pot synthesis without complex apparatus.
The choice of solvent and temperature control is critical for optimizing yield and minimizing side reactions, such as over-chlorination or decomposition.
The purification steps involving crystallization from aliphatic hydrocarbons improve product purity significantly, often achieving purities above 85% after distillation.
The reaction mechanism involves initial chlorination of the allyl isothiocyanate to form intermediates, followed by oxidative cyclization and halogenation to yield the chloromethyl thiazole.
Analytical techniques such as NMR (1H, 13C), IR spectroscopy, mass spectrometry, and HPLC are routinely used to characterize the product and confirm purity.
Análisis De Reacciones Químicas
Types of Reactions: 4-(Chloromethyl)-2-phenyl-1,3-thiazole undergoes various types of chemical reactions, including:
Nucleophilic Substitution: The chloromethyl group can be replaced by nucleophiles such as amines, thiols, and alcohols, leading to the formation of substituted thiazole derivatives.
Oxidation: The compound can be oxidized to form sulfoxides or sulfones, depending on the oxidizing agent and reaction conditions.
Reduction: Reduction of the thiazole ring can yield dihydrothiazole derivatives.
Common Reagents and Conditions:
Nucleophilic Substitution: Typical reagents include sodium azide (NaN₃), potassium thiocyanate (KSCN), and primary amines. Reactions are often carried out in polar aprotic solvents such as dimethylformamide (DMF) or dimethyl sulfoxide (DMSO) at elevated temperatures.
Oxidation: Common oxidizing agents include hydrogen peroxide (H₂O₂) and m-chloroperoxybenzoic acid (m-CPBA).
Reduction: Reducing agents such as lithium aluminum hydride (LiAlH₄) or sodium borohydride (NaBH₄) are used under anhydrous conditions.
Major Products Formed:
Nucleophilic Substitution: Substituted thiazole derivatives with various functional groups.
Oxidation: Sulfoxides and sulfones.
Reduction: Dihydrothiazole derivatives.
Aplicaciones Científicas De Investigación
Chemical Properties and Structure
- Molecular Formula : CHClN S
- SMILES : C1=CC=C(C=C1)C2=NC(=CS2)CCl
- InChIKey : SVEGSFSFMLCNFF-UHFFFAOYSA-N
The presence of the chloromethyl group allows for various chemical modifications, making it a valuable building block in synthetic chemistry.
Medicinal Chemistry Applications
4-(Chloromethyl)-2-phenyl-1,3-thiazole has been explored for its potential therapeutic applications:
-
Antimicrobial Activity : Studies have shown that derivatives of this compound exhibit significant antimicrobial properties. For instance, compounds derived from this compound have demonstrated effective inhibition against Staphylococcus aureus and Escherichia coli .
Compound Type Activity IC (µM) Thiazole Derivative Antimicrobial 0.42 - 0.80 - Anticancer Potential : Research indicates that thiazole derivatives can induce apoptosis in cancer cells. For example, a study reported that specific derivatives exhibited IC values of 5.73 µM against MCF-7 breast cancer cells, highlighting their potential as anticancer agents .
Synthetic Chemistry Applications
The compound serves as a crucial intermediate in the synthesis of more complex molecules:
- Building Block for Thiazole Derivatives : The chloromethyl group facilitates nucleophilic substitution reactions, allowing for the introduction of various functional groups. This property is exploited in the synthesis of thiazole-based scaffolds that have been evaluated for diverse biological activities .
Common Reactions
Reaction Type | Description |
---|---|
Nucleophilic Substitution | Chlorine atom replaced by nucleophiles (e.g., azides, amines) |
Oxidation | Involves reagents like potassium permanganate |
Reduction | Uses reducing agents such as lithium aluminum hydride |
Material Science Applications
Beyond medicinal uses, this compound is also being investigated for its role in developing advanced materials:
- Polymer Chemistry : The compound's reactivity allows it to be incorporated into polymers and coatings with enhanced properties. Its ability to form covalent bonds with various substrates makes it a candidate for creating functionalized materials .
Case Study 1: Antimicrobial Efficacy
A recent study evaluated the antimicrobial efficacy of several thiazole derivatives against clinical isolates. The results indicated that compounds containing the chloromethyl group exhibited higher inhibition zones compared to non-modified counterparts, suggesting enhanced interaction with bacterial membranes .
Case Study 2: Anticancer Activity
In another study involving human cancer cell lines, treatment with derivatives of this compound resulted in a dose-dependent decrease in cell viability. Flow cytometry analysis confirmed an increase in apoptotic cells following treatment, indicating its potential as an anticancer agent .
Mecanismo De Acción
The mechanism of action of 4-(Chloromethyl)-2-phenyl-1,3-thiazole involves its interaction with specific molecular targets and pathways:
Molecular Targets: The compound can interact with enzymes, receptors, and other proteins, modulating their activity and leading to various biological effects.
Pathways Involved: Depending on the specific derivative and application, the compound may influence pathways related to cell proliferation, apoptosis, and signal transduction.
Comparación Con Compuestos Similares
Comparison with Structurally Similar Thiazole Derivatives
Thiazole derivatives exhibit diverse biological activities influenced by substituent type, position, and electronic effects. Below is a detailed comparison of 4-(chloromethyl)-2-phenyl-1,3-thiazole with key analogs:
Table 1: Structural and Functional Comparison of Selected Thiazole Derivatives
Impact of Substituents on Reactivity and Bioactivity
- Chloromethyl Group : The -CH2Cl group in this compound enhances electrophilicity, enabling nucleophilic substitution reactions to form amides, sulfides, or hybrid scaffolds . In contrast, methyl (-CH3) or methoxy (-OCH3) substituents (e.g., in 4-(4-methylphenyl)-2-phenyl-1,3-thiazole) reduce reactivity .
- Aryl vs. Alkyl Substituents: Phenyl Groups: 2-Phenyl substitution (as in the lead compound) improves π-π stacking with bacterial enzyme active sites, contributing to antimicrobial activity . Halogenated Aryl Groups: Derivatives like 4-(chloromethyl)-2-(4-chlorophenyl)-1,3-thiazole exhibit stronger antibacterial effects due to increased lipophilicity and membrane disruption . Amino Groups: 2-Amino-substituted thiazoles (e.g., 5-(dichlorophenyl)-4-chlorobenzyl-thiazole) show enhanced binding to bacterial DNA gyrase .
Antimicrobial Activity Trends
- Phenyl Position : In isomers of 2-(4-hydroxyphenyl)-1,3-thiazole, the compound with the 4-hydroxyphenyl group at position 2 (vs. 4) showed lower MIC (125–150 µg/mL) against S. aureus and A. niger .
- Halogen Effects : Bromine or chlorine at the para position of the phenyl ring (e.g., 4-(4-bromophenyl)-thiazole) increases antibacterial potency compared to methyl or methoxy groups .
Actividad Biológica
4-(Chloromethyl)-2-phenyl-1,3-thiazole is a heterocyclic compound that has garnered attention for its diverse biological activities. This article provides an overview of its synthesis, biological properties, and potential therapeutic applications based on current research findings.
Chemical Structure and Properties
This compound is characterized by a thiazole ring substituted with a chloromethyl group at the fourth position and a phenyl group at the second position. Its molecular formula is with a molecular weight of approximately 209.7 g/mol. The presence of the chloromethyl group enhances its reactivity, making it a valuable intermediate in synthetic chemistry and medicinal applications.
Synthesis
The synthesis of this compound typically involves several key steps:
- Formation of the Thiazole Ring : The thiazole structure is formed through cyclization reactions involving appropriate precursors.
- Chloromethylation : The introduction of the chloromethyl group can be achieved via nucleophilic substitution reactions.
- Purification : The final product is purified using standard techniques such as recrystallization or chromatography.
Biological Activity
Research indicates that this compound exhibits significant biological activities, including:
Antimicrobial Properties
Studies have demonstrated that this compound shows effectiveness against various bacterial strains, suggesting potential applications in treating infections.
Anti-inflammatory and Anticancer Activities
Preliminary studies indicate that this compound may possess anti-inflammatory and anticancer properties. It appears to inhibit specific enzymes involved in bacterial cell wall synthesis and interfere with cellular signaling pathways related to inflammation and cancer progression.
Comparative Biological Activity
The biological activity of this compound can be compared with related compounds to highlight its unique properties:
Compound Name | Key Features | Biological Activity |
---|---|---|
2-Phenylthiazole | Lacks chloromethyl group | Less reactive |
4-Methyl-2-phenyl-1,3-thiazole | Contains methyl instead of chloromethyl | Altered reactivity |
2-Phenyl-1,3-thiazole-4-carboxylic acid | Carboxylic acid derivative | Different solubility |
4-Chlorothiazole | Similar thiazole ring without phenyl substitution | Different properties |
While specific mechanisms of action for this compound have not been fully elucidated, its electrophilic chloromethyl group suggests potential for alkylating other molecules. This reactivity could play a role in its interactions with biological systems.
Case Studies
Recent investigations have focused on the synthesis and evaluation of novel derivatives based on the thiazole framework. For instance:
Q & A
Q. Basic: What are the common synthetic routes for 4-(Chloromethyl)-2-phenyl-1,3-thiazole, and how can reaction conditions be optimized to improve yield and purity?
Answer:
The synthesis of this compound typically involves Hantzsch condensation between thiosemicarbazones and halogenated ketones (e.g., bromoacetophenone derivatives). For example, analogous thiazole compounds are synthesized via refluxing thiosemicarbazones with 2-bromo-4’-chloroacetophenone in ethanol, followed by purification via crystallization . Key optimization strategies include:
- Solvent selection : Polar aprotic solvents like DMSO or DMF enhance reaction rates but may require careful temperature control to avoid side reactions.
- Catalyst use : Acidic or basic catalysts (e.g., p-toluenesulfonic acid) can accelerate cyclization.
- Purity validation : Melting point analysis (e.g., 49–50°C for the pure compound ) and spectroscopic techniques (NMR, IR) ensure structural integrity .
Q. Basic: Which spectroscopic and chromatographic techniques are most effective for characterizing the structural integrity and purity of this compound?
Answer:
Nuclear Magnetic Resonance (NMR) is critical for confirming the thiazole ring and chloromethyl group:
- ¹H NMR : Peaks at δ 4.8–5.2 ppm (CH2Cl) and aromatic protons (δ 7.2–8.1 ppm) .
- ¹³C NMR : Signals near 40 ppm (CH2Cl) and 150–160 ppm (thiazole C-2 and C-4) .
Infrared (IR) Spectroscopy : Stretching vibrations at ~680 cm⁻¹ (C-Cl) and 1600 cm⁻¹ (C=N) confirm functional groups .
Thin-Layer Chromatography (TLC) with silica gel and ethyl acetate/hexane (1:3) monitors reaction progress .
Q. Advanced: How can computational methods like molecular docking or DFT calculations be integrated with experimental data to predict the biological activity of this compound derivatives?
Answer:
- Molecular Docking : Tools like AutoDock Vina predict binding affinities to target proteins (e.g., Leishmania enzymes). For example, thiazole derivatives showed strong interactions with trypanothione reductase, correlating with in vitro antiparasitic activity .
- Density Functional Theory (DFT) : Calculates electronic properties (HOMO-LUMO gaps) to assess reactivity. A lower gap (~4 eV) suggests potential for nucleophilic attacks, aligning with observed alkylation activity .
- Validation : Compare computational predictions with in vitro assays (e.g., IC50 values) to refine models .
Q. Advanced: What strategies are employed to resolve contradictions in reported biological activities of thiazole derivatives, and how can structure-activity relationship (SAR) studies be designed for this compound?
Answer:
Contradictions often arise from variations in substituent positioning or assay conditions. Strategies include:
- Systematic SAR : Synthesize derivatives with controlled modifications (e.g., halogenation at the phenyl ring or substitution at the thiazole C-4). For example, 4-(4-chlorophenyl) analogs showed enhanced antiparasitic activity compared to unsubstituted derivatives .
- Standardized Assays : Use uniform protocols (e.g., fixed concentrations, cell lines) to minimize variability.
- Meta-Analysis : Cross-reference crystallographic data (e.g., bond lengths from SHELX-refined structures ) with biological outcomes to identify structural determinants of activity.
Q. Basic: What are the critical safety considerations when handling this compound in laboratory settings?
Answer:
- Hazard Profile : The chloromethyl group renders the compound corrosive (causes severe skin/eye damage). Use nitrile gloves, goggles, and fume hoods .
- Storage : Store in amber glass bottles at 2–8°C to prevent degradation .
- Spill Management : Neutralize with sodium bicarbonate and adsorb using vermiculite .
Q. Advanced: How can X-ray crystallography and SHELX software be utilized to determine the crystal structure of this compound, and what challenges might arise during refinement?
Answer:
- Data Collection : Use Mo-Kα radiation (λ = 0.71073 Å) to collect intensity data. For similar thiazoles, monoclinic systems (e.g., P2₁/c) are common .
- SHELX Workflow :
- Validation : Check R-factors (< 0.05) and residual electron density (< 0.3 eÅ⁻³) .
Propiedades
IUPAC Name |
4-(chloromethyl)-2-phenyl-1,3-thiazole | |
---|---|---|
Source | PubChem | |
URL | https://pubchem.ncbi.nlm.nih.gov | |
Description | Data deposited in or computed by PubChem | |
InChI |
InChI=1S/C10H8ClNS/c11-6-9-7-13-10(12-9)8-4-2-1-3-5-8/h1-5,7H,6H2 | |
Source | PubChem | |
URL | https://pubchem.ncbi.nlm.nih.gov | |
Description | Data deposited in or computed by PubChem | |
InChI Key |
SVEGSFSFMLCNFF-UHFFFAOYSA-N | |
Source | PubChem | |
URL | https://pubchem.ncbi.nlm.nih.gov | |
Description | Data deposited in or computed by PubChem | |
Canonical SMILES |
C1=CC=C(C=C1)C2=NC(=CS2)CCl | |
Source | PubChem | |
URL | https://pubchem.ncbi.nlm.nih.gov | |
Description | Data deposited in or computed by PubChem | |
Molecular Formula |
C10H8ClNS | |
Source | PubChem | |
URL | https://pubchem.ncbi.nlm.nih.gov | |
Description | Data deposited in or computed by PubChem | |
DSSTOX Substance ID |
DTXSID90284978 | |
Record name | 4-(chloromethyl)-2-phenyl-1,3-thiazole | |
Source | EPA DSSTox | |
URL | https://comptox.epa.gov/dashboard/DTXSID90284978 | |
Description | DSSTox provides a high quality public chemistry resource for supporting improved predictive toxicology. | |
Molecular Weight |
209.70 g/mol | |
Source | PubChem | |
URL | https://pubchem.ncbi.nlm.nih.gov | |
Description | Data deposited in or computed by PubChem | |
CAS No. |
4771-31-7 | |
Record name | 4771-31-7 | |
Source | DTP/NCI | |
URL | https://dtp.cancer.gov/dtpstandard/servlet/dwindex?searchtype=NSC&outputformat=html&searchlist=40038 | |
Description | The NCI Development Therapeutics Program (DTP) provides services and resources to the academic and private-sector research communities worldwide to facilitate the discovery and development of new cancer therapeutic agents. | |
Explanation | Unless otherwise indicated, all text within NCI products is free of copyright and may be reused without our permission. Credit the National Cancer Institute as the source. | |
Record name | 4-(chloromethyl)-2-phenyl-1,3-thiazole | |
Source | EPA DSSTox | |
URL | https://comptox.epa.gov/dashboard/DTXSID90284978 | |
Description | DSSTox provides a high quality public chemistry resource for supporting improved predictive toxicology. | |
Synthesis routes and methods I
Procedure details
Synthesis routes and methods II
Procedure details
Synthesis routes and methods III
Procedure details
Synthesis routes and methods IV
Procedure details
Retrosynthesis Analysis
AI-Powered Synthesis Planning: Our tool employs the Template_relevance Pistachio, Template_relevance Bkms_metabolic, Template_relevance Pistachio_ringbreaker, Template_relevance Reaxys, Template_relevance Reaxys_biocatalysis model, leveraging a vast database of chemical reactions to predict feasible synthetic routes.
One-Step Synthesis Focus: Specifically designed for one-step synthesis, it provides concise and direct routes for your target compounds, streamlining the synthesis process.
Accurate Predictions: Utilizing the extensive PISTACHIO, BKMS_METABOLIC, PISTACHIO_RINGBREAKER, REAXYS, REAXYS_BIOCATALYSIS database, our tool offers high-accuracy predictions, reflecting the latest in chemical research and data.
Strategy Settings
Precursor scoring | Relevance Heuristic |
---|---|
Min. plausibility | 0.01 |
Model | Template_relevance |
Template Set | Pistachio/Bkms_metabolic/Pistachio_ringbreaker/Reaxys/Reaxys_biocatalysis |
Top-N result to add to graph | 6 |
Feasible Synthetic Routes
Descargo de responsabilidad e información sobre productos de investigación in vitro
Tenga en cuenta que todos los artículos e información de productos presentados en BenchChem están destinados únicamente con fines informativos. Los productos disponibles para la compra en BenchChem están diseñados específicamente para estudios in vitro, que se realizan fuera de organismos vivos. Los estudios in vitro, derivados del término latino "in vidrio", involucran experimentos realizados en entornos de laboratorio controlados utilizando células o tejidos. Es importante tener en cuenta que estos productos no se clasifican como medicamentos y no han recibido la aprobación de la FDA para la prevención, tratamiento o cura de ninguna condición médica, dolencia o enfermedad. Debemos enfatizar que cualquier forma de introducción corporal de estos productos en humanos o animales está estrictamente prohibida por ley. Es esencial adherirse a estas pautas para garantizar el cumplimiento de los estándares legales y éticos en la investigación y experimentación.