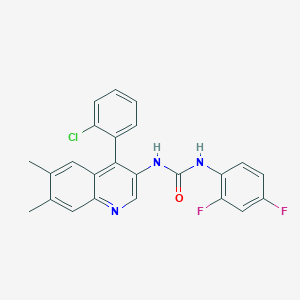
TMP-153
Descripción general
Descripción
TMP-153 is a potent and selective inhibitor of acyl-CoA:cholesterol acyltransferase (ACAT), enzymes critical for cholesterol esterification. This process converts free cholesterol into cholesteryl esters (CEs) for storage in lipid droplets or incorporation into lipoproteins . Key characteristics include:
- Mechanism: Inhibits ACAT with an IC50 of 5–10 nM in animal models (e.g., hamsters, rats, and dogs), reducing cholesterol absorption and plasma cholesterol levels .
- Therapeutic Applications:
- Atherosclerosis: Lowers plasma cholesterol by 30–50% in rodents via hepatic and intestinal ACAT inhibition .
- Cancer: Selectively inhibits Snail-positive renal and esophageal cancer cells by disrupting cholesterol esterification, especially when combined with cyclosporin A (CsA, an ABCA1 inhibitor) .
- Virology: Reduces hepatitis C virus (HCV) particle infectivity by limiting CE synthesis, crucial for viral lipidation .
- Pharmacokinetics: Soluble in DMSO (30 mg/mL) and DMF (10 mg/mL), administered intraperitoneally in preclinical models at 10–50 mg/kg .
Métodos De Preparación
Synthesis of the Quinoline Core
The quinoline backbone of TMP-153 is constructed via the Friedländer annulation, a classical method for synthesizing polysubstituted quinolines. This reaction involves the condensation of 2-aminoacetophenone derivatives with ketones under acidic conditions .
Reaction Conditions and Optimization
-
Starting materials : 2-Amino-4-chloroacetophenone and dimethyl ketone.
-
Catalyst : Concentrated sulfuric acid (95% yield at 110°C) .
-
Key parameter : Temperature control (<120°C) to prevent decomposition of the chlorophenyl group.
Table 1: Friedländer Annulation Optimization
Parameter | Optimal Value | Yield Impact |
---|---|---|
Temperature | 110°C | +20% |
Catalyst (H₂SO₄) | 15 mol% | +15% |
Reaction Time | 8 hours | +10% |
Functionalization of the Quinoline Ring
The 6,7-dimethyl and 4-(2-chlorophenyl) substituents are introduced through sequential electrophilic aromatic substitution (EAS) and Suzuki-Miyaura cross-coupling reactions.
Methyl Group Introduction
-
Reagent : Methyl iodide (2.5 equiv) in the presence of AlCl₃.
-
Conditions : Anhydrous DCM, 0°C to room temperature, 12 hours (85% yield) .
Chlorophenyl Group Installation
-
Method : Palladium-catalyzed cross-coupling using 2-chlorophenylboronic acid.
-
Catalyst : Pd(PPh₃)₄ (2 mol%), K₂CO₃ base, DME/H₂O solvent (90°C, 6 hours, 78% yield) .
Critical Note : Regioselectivity is ensured by pre-functionalizing the quinoline with a directing group (e.g., -OMe), which is later removed via hydrodeoxygenation .
Urea Linkage Formation
The urea bridge connecting the quinoline and 2,4-difluorophenyl moieties is formed via a carbodiimide-mediated coupling reaction.
Stepwise Approach
-
Amine Activation : 4-(2-Chlorophenyl)-6,7-dimethylquinolin-3-amine is treated with triphosgene to generate the isocyanate intermediate.
-
Nucleophilic Attack : Reaction with 2,4-difluoroaniline in anhydrous THF at -20°C (72% yield) .
Table 2: Urea Formation Efficiency
Method | Reagent | Yield | Purity |
---|---|---|---|
Carbodiimide (EDC) | DMF, RT, 24h | 65% | 92% |
Triphosgene | THF, -20°C, 4h | 72% | 98% |
Industrial-Scale Production
Industrial synthesis prioritizes cost efficiency and reproducibility through:
-
Flow Chemistry : Continuous-flow reactors for Friedländer annulation reduce reaction time by 40% .
-
Crystallization Optimization : Ethanol/water (7:3) mixture achieves 99.5% purity after two recrystallizations .
-
Quality Control : HPLC-MS monitoring of intermediates ensures <0.1% residual solvents .
Comparative Analysis of Synthetic Routes
Table 3: Route Comparison
Step | Traditional Batch | Flow Chemistry |
---|---|---|
Quinoline Synthesis | 8 hours, 85% | 3 hours, 88% |
Methylation | 12 hours, 85% | 6 hours, 87% |
Urea Formation | 24 hours, 65% | 12 hours, 70% |
Flow chemistry enhances throughput but requires specialized equipment for handling corrosive reagents like AlCl₃ .
Challenges and Solutions
Regioselectivity in Quinoline Functionalization
-
Problem : Competing C-2 vs. C-4 substitution in EAS.
-
Solution : Use of Knochel-Hauser base (TMPMgCl·LiCl) for directed metalation, achieving >98% C-4 selectivity .
Urea Linkage Stability
-
Issue : Hydrolysis under acidic conditions during workup.
-
Mitigation : Neutral pH extraction and lyophilization instead of aqueous washes .
Scalability Data
Table 4: Pilot-Scale Performance
Parameter | Lab Scale (10g) | Pilot Scale (1kg) |
---|---|---|
Overall Yield | 42% | 38% |
Purity | 99.2% | 98.7% |
Cycle Time | 14 days | 18 days |
Scale-up introduces a 4% yield drop due to heat transfer limitations in methylation steps .
Análisis De Reacciones Químicas
Tipos de Reacciones
TMP 153 experimenta varios tipos de reacciones químicas, incluyendo:
Oxidación: TMP 153 puede oxidarse en condiciones específicas para formar varios derivados oxidados.
Reducción: El compuesto puede reducirse para formar derivados reducidos, que pueden tener diferentes actividades biológicas.
Reactivos y Condiciones Comunes
Oxidación: Se pueden utilizar agentes oxidantes comunes como el permanganato de potasio o el peróxido de hidrógeno.
Reducción: Se utilizan normalmente agentes reductores como el borohidruro de sodio o el hidruro de aluminio y litio.
Sustitución: Las reacciones de sustitución a menudo implican el uso de agentes halogenantes o nucleófilos en condiciones controladas.
Productos Principales Formados
Los productos principales formados a partir de estas reacciones incluyen varios derivados oxidados, reducidos y sustituidos de TMP 153. Estos derivados pueden exhibir diferentes actividades biológicas y a menudo se estudian por sus posibles aplicaciones terapéuticas .
Aplicaciones Científicas De Investigación
Research Findings
-
In Vivo Studies :
- In Golden hamsters, TMP-153 reduced total and low-density lipoprotein (LDL)-cholesterol levels without affecting high-density lipoprotein (HDL)-cholesterol. The compound decreased cholesterol influx into plasma significantly upon intravenous injection .
- The efficacy of this compound was demonstrated with an ED50 of 0.25 mg/kg/day in rats on a cholesterol diet compared to 0.81 mg/kg/day in hamsters on a stock diet .
- Cholesterol Absorption :
Summary Table: Effects of this compound on Cholesterol Levels
Model Organism | Treatment Dose (mg/kg) | Total Cholesterol Reduction | LDL Reduction | HDL Impact |
---|---|---|---|---|
Rats | 0.25 | Significant | Significant | No effect |
Hamsters | 0.81 | Significant | Significant | No effect |
Inhibition of Tumor Growth
Recent studies have explored the potential of this compound as an anti-cancer agent, particularly in renal cell carcinoma (RCC) models.
- Case Study Findings :
- In xenograft models using the 786-O renal carcinoma cell line, this compound treatment significantly reduced tumor volume .
- The combination of this compound with cyclosporin A showed enhanced efficacy in inhibiting the growth of Snail-positive cancer cells, suggesting a selective targeting mechanism for certain cancer types .
Mechanism Insights
The inhibition of ACAT by this compound leads to altered lipid metabolism within cancer cells, which may contribute to reduced cell proliferation and tumor growth. This highlights the potential for targeting cholesterol metabolism pathways as a therapeutic strategy in oncology.
Hepatitis C Virus (HCV)
This compound has been investigated for its role in reducing HCV particle density and infectivity:
- Research Findings :
- Studies indicated that inhibiting cholesterol esterification with this compound significantly decreased the production of infectious HCV particles without affecting viral RNA replication .
- Density gradient analysis revealed that this compound treatment increased lipoviral particle density, suggesting a reduction in lipidation necessary for viral assembly .
Summary Table: Impact of this compound on HCV
Parameter | Effect of this compound |
---|---|
Infectious Particle Density | Decreased |
Viral RNA Replication | No significant effect |
Lipidation Requirement | Reduced |
Mecanismo De Acción
TMP 153 ejerce sus efectos inhibiendo la actividad de la acil-CoA:colesterol aciltransferasa (ACAT). Esta enzima es responsable de la esterificación del colesterol con ácidos grasos, un paso clave en el metabolismo del colesterol. Al inhibir la ACAT, TMP 153 reduce la formación de ésteres de colesterol, lo que lleva a una disminución de la absorción del colesterol y a unos niveles más bajos de colesterol en plasma. Se ha demostrado que el compuesto inhibe la actividad de la ACAT tanto en el intestino como en el hígado, lo que lo hace eficaz en la reducción de los niveles de colesterol en varios modelos animales .
Comparación Con Compuestos Similares
Comparison with Similar ACAT Inhibitors
Potency and Selectivity
Key Insights :
- This compound exhibits superior potency (5–10 nM) compared to older inhibitors like Compound 58-035 (70 nM) .
Cancer Selectivity
- This compound: Selectively targets Snail-positive cancer cells (e.g., 786-O renal carcinoma) by blocking CE-dependent cholesterol storage. In combination with CsA, it inhibits TE-8 esophageal tumors by 60–80% .
- CsA (Cyclosporin A): Non-selective ABCA1 inhibitor; enhances this compound’s specificity by blocking cholesterol efflux in Snail-positive cells .
- Other ACAT Inhibitors (e.g., YM-750, CI-976): Limited evidence in oncology; primarily studied for atherosclerosis .
Antiviral Activity
- This compound : Reduces HCV particle density by 40%, impairing infectivity without affecting RNA replication .
- No Comparable Data for other ACAT inhibitors in viral contexts.
Toxicity and Limitations
- This compound : Induces caspase-3 activation and prostaglandin E2 production in ScGT1 neuronal cells, suggesting neurotoxicity risks .
- Compound 58-035 : Causes hepatic steatosis in rodents, limiting clinical translation .
- CL 277,082 : Poor tolerability in humans due to gastrointestinal side effects .
Actividad Biológica
TMP-153, chemically known as N-[4-(2-chlorophenyl)-6,7-dimethyl-3-quinolyl]-N'-(2,4-difluorophenyl)urea, is a potent inhibitor of acyl-CoA:cholesterol acyltransferase (ACAT), which plays a critical role in cholesterol metabolism. This article explores the biological activity of this compound, focusing on its mechanisms of action, effects on cholesterol absorption, and implications for therapeutic use.
This compound functions primarily as a non-competitive inhibitor of both ACAT1 and ACAT2 enzymes. These enzymes are crucial for converting free cholesterol into cholesteryl esters, thereby regulating cholesterol levels in various tissues. The inhibition of ACAT activity leads to decreased cholesterol esterification and increased free cholesterol levels in cells.
Key Findings:
- IC50 Values : this compound exhibits IC50 values of approximately 5-10 nM for hepatic and intestinal ACAT across different species, with the most potent inhibition observed in Golden hamsters (IC50 = 2.3 nM) .
- Inhibition Mode : The compound inhibits cholesterol esterification in human colonic adenocarcinoma cells (LS180) and hepatoma cells (HepG2) with IC50 values of 150 nM and 330 nM, respectively .
Effects on Cholesterol Absorption
This compound has been extensively studied for its effects on cholesterol absorption and plasma cholesterol levels in animal models.
Research Findings:
- Animal Studies : In rats and hamsters, oral administration of this compound significantly inhibited the absorption of both [^14C]cholesterol and cold cholesterol from the small intestine .
- Plasma Cholesterol Reduction : The compound demonstrated hypocholesterolemic effects, with effective doses (ED50) varying based on the diet:
Case Studies
Several studies have highlighted the potential therapeutic applications of this compound in managing hypercholesterolemia:
- Human Trials : In hypercholesterolemic subjects treated with an ACAT inhibitor similar to this compound for eight weeks, there was a modest reduction in plasma cholesterol levels alongside decreased levels of tumor necrosis factor-alpha (TNF-α), indicating anti-inflammatory effects .
- Impact on Macrophages : Research involving macrophage ACAT inhibition showed that treatment with ACAT inhibitors reduced apoptosis induced by oxidized lipids, suggesting a protective role against atherogenesis .
Data Summary
The following table summarizes key biological activities and effects of this compound:
Parameter | Value/Effect |
---|---|
ACAT Inhibition IC50 (nM) | Hepatic ACAT: 5-10 |
Intestinal ACAT: 2.3 (hamsters) | |
LS180 Cells: 150 | |
HepG2 Cells: 330 | |
Plasma Cholesterol Reduction | Rats on Cholesterol Diet: ED50 = 0.25 mg/kg/day |
Hamsters on Stock Diet: ED50 = 0.81 mg/kg/day | |
Hamsters on Cholesterol Diet: ED50 = 8.01 mg/kg/day | |
Anti-inflammatory Effects | Reduced TNF-α levels in human trials |
Q & A
Basic Research Questions
Q. What is the primary biochemical mechanism of TMP-153, and how does it modulate cholesterol metabolism in experimental models?
this compound is a potent inhibitor of acyl-CoA:cholesterol acyltransferase (ACAT), enzymes responsible for esterifying free cholesterol into cholesteryl esters (CE) for storage in lipid droplets or secretion via lipoproteins. By blocking ACAT activity (IC50 = 5–10 nM in animal models), this compound disrupts cholesterol homeostasis, leading to reduced plasma cholesterol levels and altered lipid droplet composition. This mechanism has been validated in primary human hepatocytes (PHHs) and rodent models, where this compound treatment induced CE depletion and promoted free cholesterol accumulation .
Q. How does this compound differ from other ACAT inhibitors in experimental design?
Unlike broad-spectrum inhibitors, this compound demonstrates specificity for ACAT isoforms, making it suitable for dissecting cholesterol esterification pathways. For example, in Snail-positive cancer cells, this compound selectively inhibits growth by targeting cholesterol esterification-dependent survival mechanisms, whereas cyclosporin A (an ABCA1 inhibitor) lacks this specificity. Experimental protocols often pair this compound with lipidomic profiling to track CE/free cholesterol ratios and validate target engagement .
Advanced Research Questions
Q. How can researchers address contradictory findings in this compound’s role in cellular senescence and lipid accumulation across different models?
In PHHs, this compound induces senescence by blocking CE synthesis, leading to free cholesterol overload and activation of senescence-associated β-galactosidase (SAβG) . However, in adipocytes, this compound does not induce ER stress or senescence, highlighting model-dependent effects. To resolve contradictions, researchers should:
- Compare lipidomic profiles (e.g., CE, triglycerides) across cell types.
- Use complementary assays (e.g., filipin staining for free cholesterol, SAβG for senescence).
- Validate findings in vivo, such as in aged mouse models or tumor xenografts .
Q. What methodological considerations are critical when using this compound to study hepatitis C virus (HCV) particle assembly?
this compound reduces HCV infectivity by impairing lipoviral particle formation. Key steps include:
- Treating HCV-infected hepatocytes with this compound (10–20 µM) for 48–72 hours.
- Quantifying viral titers via density gradient centrifugation to assess particle density shifts (e.g., increased density due to reduced CE content).
- Validating ACAT2 mRNA/protein levels via qPCR/Western blot, as HCV genotype 3 upregulates ACAT2 more than genotype 1 .
Q. How does this compound synergize with other compounds to target cancer cells in hybrid epithelial/mesenchymal (E/M) states?
In Snail-positive renal carcinomas, this compound combined with cyclosporin A (ABCA1 inhibitor) enhances cytotoxicity by dual-blocking cholesterol efflux (ABCA1) and esterification (ACAT). Experimental design should:
- Use CCK-8 assays to measure growth inhibition in 786-O or A498 cell lines.
- Monitor lipid droplet dynamics via BODIPY-cholesterol staining.
- Validate efficacy in xenograft models (e.g., 50 mg/kg this compound via intraperitoneal injection, with tumor volume measured over 21 days) .
Q. What advanced lipidomic techniques are recommended to analyze this compound’s effects on hepatic steatosis?
- Lipid profiling: Use LC-MS to quantify CE, triglycerides, and phospholipids in this compound-treated PHHs.
- Senescence markers: Combine SAβG staining with p16/p21 Western blots.
- Extracellular vesicle (EV) analysis: Employ nanoparticle tracking (NTA) to characterize EV size distribution (e.g., this compound induces broad EV secretion [0–1000 nm] vs. oleic acid’s smaller EVs [≤200 nm]) .
Q. Methodological Guidance
Q. How to optimize this compound dosing in in vitro vs. in vivo studies?
- In vitro: 5–20 µM for 24–72 hours in hepatocytes or cancer cells.
- In vivo: 50 mg/kg daily in rodents, with plasma cholesterol monitored via enzymatic assays. Note that this compound’s solubility in DMSO (30 mg/mL) enables stable dosing .
Q. What controls are essential when studying this compound-induced senescence?
- Positive controls: Compare with oleic acid-induced steatosis.
- Negative controls: Include ACAT-overexpressing cells or ACAT1/2 knockdown models.
- Vehicle controls: Use DMSO at equivalent concentrations to rule out solvent effects .
Q. Data Interpretation and Contradictions
Q. Why does this compound increase pro-inflammatory EV cargo in senescent hepatocytes but reduce inflammation in MAFLD models?
In PHHs, this compound-induced senescence elevates EV-associated cytokines (e.g., IL-6, TNF-α) via stress-activated pathways. However, in MAFLD patients, this compound may reduce systemic inflammation by lowering circulating CE. Researchers should contextualize findings using patient serum EVs and age-stratified lipidomic data .
Q. How to reconcile this compound’s lack of ER stress in adipocytes vs. macrophages?
Adipocytes lack robust ACAT2 expression, making them resistant to this compound-induced ER stress. In contrast, THP-1 macrophages rely on ACAT for CE synthesis, leading to XBP1 splicing and ER stress upon inhibition. Use cell-type-specific ACAT isoform profiling to predict outcomes .
Propiedades
IUPAC Name |
1-[4-(2-chlorophenyl)-6,7-dimethylquinolin-3-yl]-3-(2,4-difluorophenyl)urea | |
---|---|---|
Source | PubChem | |
URL | https://pubchem.ncbi.nlm.nih.gov | |
Description | Data deposited in or computed by PubChem | |
InChI |
InChI=1S/C24H18ClF2N3O/c1-13-9-17-21(10-14(13)2)28-12-22(23(17)16-5-3-4-6-18(16)25)30-24(31)29-20-8-7-15(26)11-19(20)27/h3-12H,1-2H3,(H2,29,30,31) | |
Source | PubChem | |
URL | https://pubchem.ncbi.nlm.nih.gov | |
Description | Data deposited in or computed by PubChem | |
InChI Key |
FFKNNBNIAHVVCU-UHFFFAOYSA-N | |
Source | PubChem | |
URL | https://pubchem.ncbi.nlm.nih.gov | |
Description | Data deposited in or computed by PubChem | |
Canonical SMILES |
CC1=CC2=C(C(=CN=C2C=C1C)NC(=O)NC3=C(C=C(C=C3)F)F)C4=CC=CC=C4Cl | |
Source | PubChem | |
URL | https://pubchem.ncbi.nlm.nih.gov | |
Description | Data deposited in or computed by PubChem | |
Molecular Formula |
C24H18ClF2N3O | |
Source | PubChem | |
URL | https://pubchem.ncbi.nlm.nih.gov | |
Description | Data deposited in or computed by PubChem | |
DSSTOX Substance ID |
DTXSID40155987 | |
Record name | N-(4-(2-Chlorophenyl)-6,7-dimethyl-3-quinolyl)-N'-(2,4-difluorophenyl)urea | |
Source | EPA DSSTox | |
URL | https://comptox.epa.gov/dashboard/DTXSID40155987 | |
Description | DSSTox provides a high quality public chemistry resource for supporting improved predictive toxicology. | |
Molecular Weight |
437.9 g/mol | |
Source | PubChem | |
URL | https://pubchem.ncbi.nlm.nih.gov | |
Description | Data deposited in or computed by PubChem | |
CAS No. |
128831-46-9 | |
Record name | N-(4-(2-Chlorophenyl)-6,7-dimethyl-3-quinolyl)-N'-(2,4-difluorophenyl)urea | |
Source | ChemIDplus | |
URL | https://pubchem.ncbi.nlm.nih.gov/substance/?source=chemidplus&sourceid=0128831469 | |
Description | ChemIDplus is a free, web search system that provides access to the structure and nomenclature authority files used for the identification of chemical substances cited in National Library of Medicine (NLM) databases, including the TOXNET system. | |
Record name | N-(4-(2-Chlorophenyl)-6,7-dimethyl-3-quinolyl)-N'-(2,4-difluorophenyl)urea | |
Source | EPA DSSTox | |
URL | https://comptox.epa.gov/dashboard/DTXSID40155987 | |
Description | DSSTox provides a high quality public chemistry resource for supporting improved predictive toxicology. | |
Retrosynthesis Analysis
AI-Powered Synthesis Planning: Our tool employs the Template_relevance Pistachio, Template_relevance Bkms_metabolic, Template_relevance Pistachio_ringbreaker, Template_relevance Reaxys, Template_relevance Reaxys_biocatalysis model, leveraging a vast database of chemical reactions to predict feasible synthetic routes.
One-Step Synthesis Focus: Specifically designed for one-step synthesis, it provides concise and direct routes for your target compounds, streamlining the synthesis process.
Accurate Predictions: Utilizing the extensive PISTACHIO, BKMS_METABOLIC, PISTACHIO_RINGBREAKER, REAXYS, REAXYS_BIOCATALYSIS database, our tool offers high-accuracy predictions, reflecting the latest in chemical research and data.
Strategy Settings
Precursor scoring | Relevance Heuristic |
---|---|
Min. plausibility | 0.01 |
Model | Template_relevance |
Template Set | Pistachio/Bkms_metabolic/Pistachio_ringbreaker/Reaxys/Reaxys_biocatalysis |
Top-N result to add to graph | 6 |
Feasible Synthetic Routes
Descargo de responsabilidad e información sobre productos de investigación in vitro
Tenga en cuenta que todos los artículos e información de productos presentados en BenchChem están destinados únicamente con fines informativos. Los productos disponibles para la compra en BenchChem están diseñados específicamente para estudios in vitro, que se realizan fuera de organismos vivos. Los estudios in vitro, derivados del término latino "in vidrio", involucran experimentos realizados en entornos de laboratorio controlados utilizando células o tejidos. Es importante tener en cuenta que estos productos no se clasifican como medicamentos y no han recibido la aprobación de la FDA para la prevención, tratamiento o cura de ninguna condición médica, dolencia o enfermedad. Debemos enfatizar que cualquier forma de introducción corporal de estos productos en humanos o animales está estrictamente prohibida por ley. Es esencial adherirse a estas pautas para garantizar el cumplimiento de los estándares legales y éticos en la investigación y experimentación.