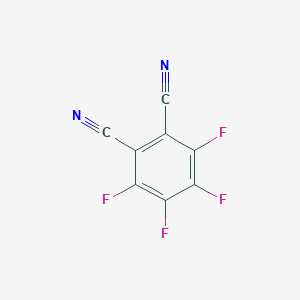
Tetrafluoroftalonitrilo
Descripción general
Descripción
Tetrafluorophthalonitrile (TFPN) is an organic compound with the chemical formula C8F4N2. It is a colorless liquid with a boiling point of 84 °C and a melting point of -86 °C. It is a highly fluorinated derivative of phthalonitrile, and is used as a reagent in organic synthesis. It is also used as a solvent for various organic compounds, as well as a catalyst for various chemical reactions.
Aplicaciones Científicas De Investigación
Síntesis de dímeros de subftalocianina dicloro
Tetrafluoroftalonitrilo se ha utilizado en la síntesis de dímeros de subftalocianina dicloro, que son compuestos con posibles aplicaciones en ciencia de materiales y química debido a sus propiedades electrónicas y ópticas únicas .
Marcos orgánicos covalentes (COFs)
Este compuesto se puede utilizar en la ingeniería de COFs con diferentes grupos funcionales. Los COFs tienen características porosas distintas, lo que los hace adecuados para aplicaciones en catálisis, optoelectrónica, energía y ciencias ambientales .
Materiales electrocrómicos
La influencia del acoplamiento intermolecular en el transporte de electrones e iones en películas delgadas de ftalocianina sustituidas de manera diferente se ha estudiado como materiales electrocrómicos. Esta es una aplicación de química que explora las condiciones óptimas para las propiedades electrónicas .
Mecanismo De Acción
Target of Action
Tetrafluorophthalonitrile is a chemical compound with the formula
C6F4(CN)2C_6F_4(CN)_2C6F4(CN)2
. It is primarily used in the synthesis of other compounds, such as copper (II) hexadecafluorophthalocyanine . The primary targets of Tetrafluorophthalonitrile are therefore the reactants in these synthesis reactions, such as copper, copper (I) chloride, or copper (II) chloride .Mode of Action
Tetrafluorophthalonitrile interacts with its targets through chemical reactions. For example, it reacts with copper, copper (I) chloride, or copper (II) chloride to yield copper (II) hexadecafluorophthalocyanine . This reaction changes the structure of the target molecules and results in the formation of a new compound.
Biochemical Pathways
It is known that the compound plays a role in the synthesis of other compounds, such as copper (ii) hexadecafluorophthalocyanine . This suggests that Tetrafluorophthalonitrile may be involved in the biochemical pathways related to the synthesis and metabolism of these compounds.
Pharmacokinetics
The compound’s molecular weight of 20009 suggests that it may have certain ADME (Absorption, Distribution, Metabolism, and Excretion) properties For example, its relatively low molecular weight may enhance its absorption and distribution within the body
Result of Action
The primary result of Tetrafluorophthalonitrile’s action is the synthesis of other compounds, such as copper (II) hexadecafluorophthalocyanine . This compound has various applications, including use in the production of dyes and pigments. Therefore, the molecular and cellular effects of Tetrafluorophthalonitrile’s action are largely dependent on the specific compounds it helps to synthesize.
Safety and Hazards
Direcciones Futuras
Propiedades
IUPAC Name |
3,4,5,6-tetrafluorobenzene-1,2-dicarbonitrile | |
---|---|---|
Source | PubChem | |
URL | https://pubchem.ncbi.nlm.nih.gov | |
Description | Data deposited in or computed by PubChem | |
InChI |
InChI=1S/C8F4N2/c9-5-3(1-13)4(2-14)6(10)8(12)7(5)11 | |
Source | PubChem | |
URL | https://pubchem.ncbi.nlm.nih.gov | |
Description | Data deposited in or computed by PubChem | |
InChI Key |
OFLRJMBSWDXSPG-UHFFFAOYSA-N | |
Source | PubChem | |
URL | https://pubchem.ncbi.nlm.nih.gov | |
Description | Data deposited in or computed by PubChem | |
Canonical SMILES |
C(#N)C1=C(C(=C(C(=C1F)F)F)F)C#N | |
Source | PubChem | |
URL | https://pubchem.ncbi.nlm.nih.gov | |
Description | Data deposited in or computed by PubChem | |
Molecular Formula |
C8F4N2 | |
Source | PubChem | |
URL | https://pubchem.ncbi.nlm.nih.gov | |
Description | Data deposited in or computed by PubChem | |
DSSTOX Substance ID |
DTXSID6075142 | |
Record name | Tetrafluorophthalonitrile | |
Source | EPA DSSTox | |
URL | https://comptox.epa.gov/dashboard/DTXSID6075142 | |
Description | DSSTox provides a high quality public chemistry resource for supporting improved predictive toxicology. | |
Molecular Weight |
200.09 g/mol | |
Source | PubChem | |
URL | https://pubchem.ncbi.nlm.nih.gov | |
Description | Data deposited in or computed by PubChem | |
CAS No. |
1835-65-0 | |
Record name | 3,4,5,6-Tetrafluoro-1,2-benzenedicarbonitrile | |
Source | CAS Common Chemistry | |
URL | https://commonchemistry.cas.org/detail?cas_rn=1835-65-0 | |
Description | CAS Common Chemistry is an open community resource for accessing chemical information. Nearly 500,000 chemical substances from CAS REGISTRY cover areas of community interest, including common and frequently regulated chemicals, and those relevant to high school and undergraduate chemistry classes. This chemical information, curated by our expert scientists, is provided in alignment with our mission as a division of the American Chemical Society. | |
Explanation | The data from CAS Common Chemistry is provided under a CC-BY-NC 4.0 license, unless otherwise stated. | |
Record name | 3,4,5,6-Tetrafluorobenzene-1,2-dicarbonitrile | |
Source | ChemIDplus | |
URL | https://pubchem.ncbi.nlm.nih.gov/substance/?source=chemidplus&sourceid=0001835650 | |
Description | ChemIDplus is a free, web search system that provides access to the structure and nomenclature authority files used for the identification of chemical substances cited in National Library of Medicine (NLM) databases, including the TOXNET system. | |
Record name | Tetrafluorophthalonitrile | |
Source | EPA DSSTox | |
URL | https://comptox.epa.gov/dashboard/DTXSID6075142 | |
Description | DSSTox provides a high quality public chemistry resource for supporting improved predictive toxicology. | |
Record name | 3,4,5,6-tetrafluorobenzene-1,2-dicarbonitrile | |
Source | European Chemicals Agency (ECHA) | |
URL | https://echa.europa.eu/substance-information/-/substanceinfo/100.015.819 | |
Description | The European Chemicals Agency (ECHA) is an agency of the European Union which is the driving force among regulatory authorities in implementing the EU's groundbreaking chemicals legislation for the benefit of human health and the environment as well as for innovation and competitiveness. | |
Explanation | Use of the information, documents and data from the ECHA website is subject to the terms and conditions of this Legal Notice, and subject to other binding limitations provided for under applicable law, the information, documents and data made available on the ECHA website may be reproduced, distributed and/or used, totally or in part, for non-commercial purposes provided that ECHA is acknowledged as the source: "Source: European Chemicals Agency, http://echa.europa.eu/". Such acknowledgement must be included in each copy of the material. ECHA permits and encourages organisations and individuals to create links to the ECHA website under the following cumulative conditions: Links can only be made to webpages that provide a link to the Legal Notice page. | |
Retrosynthesis Analysis
AI-Powered Synthesis Planning: Our tool employs the Template_relevance Pistachio, Template_relevance Bkms_metabolic, Template_relevance Pistachio_ringbreaker, Template_relevance Reaxys, Template_relevance Reaxys_biocatalysis model, leveraging a vast database of chemical reactions to predict feasible synthetic routes.
One-Step Synthesis Focus: Specifically designed for one-step synthesis, it provides concise and direct routes for your target compounds, streamlining the synthesis process.
Accurate Predictions: Utilizing the extensive PISTACHIO, BKMS_METABOLIC, PISTACHIO_RINGBREAKER, REAXYS, REAXYS_BIOCATALYSIS database, our tool offers high-accuracy predictions, reflecting the latest in chemical research and data.
Strategy Settings
Precursor scoring | Relevance Heuristic |
---|---|
Min. plausibility | 0.01 |
Model | Template_relevance |
Template Set | Pistachio/Bkms_metabolic/Pistachio_ringbreaker/Reaxys/Reaxys_biocatalysis |
Top-N result to add to graph | 6 |
Feasible Synthetic Routes
A: Tetrafluorophthalonitrile (TFPN) has the molecular formula C8F4N2 and a molecular weight of 216.09 g/mol. [, , , ]
ANone: TFPN can be characterized using various spectroscopic techniques including:
- 19F NMR Spectroscopy: Provides information about the fluorine atoms in TFPN. [, , , ]
- 1H NMR Spectroscopy: Useful for analyzing any remaining hydrogen atoms after derivatization reactions. [, , , ]
- IR Spectroscopy: Provides information about functional groups, particularly the nitrile (C≡N) group. [, , ]
- UV-Vis Spectroscopy: Useful for studying the electronic transitions of TFPN and its derivatives, particularly phthalocyanines. [, , , , ]
- Mass Spectrometry: Confirms the molecular weight and provides information about fragmentation patterns. [, ]
A: Yes, TFPN generally shows good solubility in common organic solvents like dimethylformamide (DMF), tetrahydrofuran (THF), and diethyl ether. [, , ]
A: While TFPN itself is not a catalyst, it serves as a crucial precursor for synthesizing phthalocyanines, some of which demonstrate catalytic activity in reactions like sulfide oxidation. []
A: Yes, experimental and theoretical electron density distribution studies have been performed on TFPN and its isomer, tetrafluoroisophthalonitrile, using high-level ab initio and DFT calculations. [] These studies provided insights into the electronic properties and intermolecular interactions of these compounds.
A: Replacing fluorine atoms in TFPN with other substituents significantly influences its reactivity. For example, electron-donating groups enhance the nucleophilic aromatic substitution reactions, allowing for controlled synthesis of specific derivatives. [, , , ]
ANone: TFPN serves as a key building block for synthesizing various compounds, including:
- Phthalocyanines: TFPN is a versatile precursor for synthesizing a wide range of phthalocyanines with tailored properties. [, , , , , , , ] These phthalocyanines have applications in areas such as:
- Covalent Organic Frameworks (COFs): TFPN can be reacted with specific linkers to create porous, crystalline COFs with high chemical stability. [] These materials have potential applications in areas like gas storage and separation.
- Other Applications: TFPN has also been explored in the synthesis of various other compounds, including:
ANone: While the provided research papers do not directly address the environmental impact of TFPN, it is essential to consider the potential environmental implications of TFPN and its derivatives, especially considering the persistence of fluorinated compounds in the environment. Further research is needed to understand the degradation pathways and ecotoxicological effects of TFPN.
A: Yes, other substituted phthalonitriles, such as tetrachlorophthalonitrile, 4,5-dichlorophthalonitrile, and 4,5-difluorophthalonitrile, can be used as alternatives to TFPN. [, ] The choice of starting material depends on the desired properties of the final product.
Descargo de responsabilidad e información sobre productos de investigación in vitro
Tenga en cuenta que todos los artículos e información de productos presentados en BenchChem están destinados únicamente con fines informativos. Los productos disponibles para la compra en BenchChem están diseñados específicamente para estudios in vitro, que se realizan fuera de organismos vivos. Los estudios in vitro, derivados del término latino "in vidrio", involucran experimentos realizados en entornos de laboratorio controlados utilizando células o tejidos. Es importante tener en cuenta que estos productos no se clasifican como medicamentos y no han recibido la aprobación de la FDA para la prevención, tratamiento o cura de ninguna condición médica, dolencia o enfermedad. Debemos enfatizar que cualquier forma de introducción corporal de estos productos en humanos o animales está estrictamente prohibida por ley. Es esencial adherirse a estas pautas para garantizar el cumplimiento de los estándares legales y éticos en la investigación y experimentación.