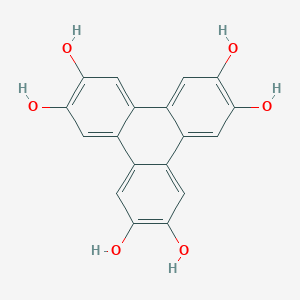
2,3,6,7,10,11-Hexahidroxitrifenileno
Descripción general
Descripción
2,3,6,7,10,11-Hexahydroxytriphenylene is an organic compound with the molecular formula C18H12O6. It is characterized by its hexahydroxy substitution on the triphenylene core, which imparts unique chemical and physical properties. This compound is notable for its applications in the synthesis of metal-organic frameworks (MOFs) and its role in various scientific research fields .
Synthetic Routes and Reaction Conditions:
Electroorganic Synthesis: One efficient method involves the anodic treatment of catechol ketals followed by acidic hydrolysis. This process is conducted in propylene carbonate, avoiding the use of toxic and expensive acetonitrile.
Ultrasound-Assisted Synthesis: Another method uses ultrasound to react catechol with ferric chloride (III) hexahydrate.
Industrial Production Methods: The industrial production of 2,3,6,7,10,11-Hexahydroxytriphenylene typically involves the oxidative trimerization of catechol derivatives using metal salts in high oxidation states, such as molybdenum pentachloride. Electrochemical methods are also employed to avoid the formation of metal waste and to achieve higher yields .
Types of Reactions:
Oxidation: The compound undergoes oxidative trimerization, which is a key step in its synthesis.
Substitution: It can participate in substitution reactions, particularly involving its hydroxyl groups.
Common Reagents and Conditions:
Oxidative Trimerization: Metal salts like molybdenum pentachloride are commonly used.
Electrochemical Oxidation: Conducted in propylene carbonate with tetrabutylammonium tetrafluoroborate as the electrolyte.
Major Products:
Aplicaciones Científicas De Investigación
2,3,6,7,10,11-Hexahydroxytriphenylene is widely used in various scientific research fields:
Biology and Medicine:
Mecanismo De Acción
Target of Action
The primary target of 2,3,6,7,10,11-Hexahydroxytriphenylene is the formation of Metal-Organic Frameworks (MOFs) . MOFs are materials formed by the coordination of metal ions and organic ligands .
Mode of Action
2,3,6,7,10,11-Hexahydroxytriphenylene interacts with its targets through coordination bonds . This interaction results in the formation of MOFs, which have a high porosity, large specific surface area, adjustable pore structure, and excellent structural stability .
Biochemical Pathways
The compound affects the pathway of MOF formation. The resulting MOFs have downstream effects in various applications, especially in the field of chemical energy storage .
Pharmacokinetics
Its solubility in tetrahydrofuran is known to be very slight .
Result of Action
The molecular and cellular effects of 2,3,6,7,10,11-Hexahydroxytriphenylene’s action are primarily observed in the formation of MOFs. These MOFs have a wide range of applications, including the enhancement of liquid crystal displays’ viewing angles .
Action Environment
The action, efficacy, and stability of 2,3,6,7,10,11-Hexahydroxytriphenylene can be influenced by environmental factors. For instance, it should be stored under an inert atmosphere at room temperature . It should also be kept away from air . Furthermore, the compound is stable and forms a tris-semiquinone monoradical upon storage for several months .
Análisis Bioquímico
Biochemical Properties
2,3,6,7,10,11-Hexahydroxytriphenylene plays a significant role in biochemical reactions, particularly in the formation of metal-organic frameworks. These frameworks are formed through the coordination of metal ions with organic ligands, such as 2,3,6,7,10,11-Hexahydroxytriphenylene. The hydroxyl groups on the compound facilitate strong interactions with metal ions, leading to the formation of stable and porous structures . Additionally, 2,3,6,7,10,11-Hexahydroxytriphenylene has been shown to interact with various enzymes and proteins, potentially influencing their activity and stability .
Cellular Effects
The effects of 2,3,6,7,10,11-Hexahydroxytriphenylene on cellular processes have been studied in various cell lines. It has been observed to exhibit cytotoxic effects on human cancer cell lines, including glioma and lung cancer cells . The compound induces cell death through mechanisms such as DNA fragmentation and apoptosis, as evidenced by increased percentages of annexin V-PI-positive cells
Molecular Mechanism
At the molecular level, 2,3,6,7,10,11-Hexahydroxytriphenylene exerts its effects through various mechanisms. One key mechanism involves the formation of stable tris-semiquinone monoradicals, which are generated through oxidation . These radicals can interact with biomolecules, leading to changes in their structure and function. Additionally, the hydroxyl groups on 2,3,6,7,10,11-Hexahydroxytriphenylene enable it to form hydrogen bonds with enzymes and proteins, potentially inhibiting or activating their activity .
Temporal Effects in Laboratory Settings
In laboratory settings, the effects of 2,3,6,7,10,11-Hexahydroxytriphenylene have been observed to change over time. The compound is relatively stable, with its tris-semiquinone monoradicals remaining stable for months . Prolonged exposure to environmental factors such as light and air may lead to degradation. Long-term studies have shown that 2,3,6,7,10,11-Hexahydroxytriphenylene can have sustained cytotoxic effects on cancer cells, indicating its potential for therapeutic applications .
Dosage Effects in Animal Models
The effects of 2,3,6,7,10,11-Hexahydroxytriphenylene vary with different dosages in animal models. At lower doses, the compound may exhibit minimal toxicity and therapeutic effects. At higher doses, it can induce toxic effects, including oxidative stress and damage to cellular components Threshold effects have been observed, where a certain dosage is required to achieve significant cytotoxicity in cancer cells
Metabolic Pathways
2,3,6,7,10,11-Hexahydroxytriphenylene is involved in various metabolic pathways, particularly those related to its role in metal-organic frameworks. The compound interacts with enzymes and cofactors involved in the synthesis and degradation of these frameworks . Additionally, its hydroxyl groups may undergo metabolic modifications, such as glucuronidation and sulfation, which can influence its bioavailability and activity .
Transport and Distribution
Within cells and tissues, 2,3,6,7,10,11-Hexahydroxytriphenylene is transported and distributed through interactions with transporters and binding proteins. These interactions facilitate its localization to specific cellular compartments, where it can exert its effects . The compound’s distribution is influenced by its physicochemical properties, such as solubility and stability, which determine its ability to penetrate cellular membranes and accumulate in target tissues .
Subcellular Localization
The subcellular localization of 2,3,6,7,10,11-Hexahydroxytriphenylene is determined by its interactions with targeting signals and post-translational modifications. The compound may be directed to specific organelles, such as the mitochondria or nucleus, where it can influence cellular processes . Its hydroxyl groups enable it to form hydrogen bonds with various biomolecules, affecting its activity and function within different subcellular compartments .
Comparación Con Compuestos Similares
2,3,6,7,10,11-Hexamethoxytriphenylene: Similar in structure but with methoxy groups instead of hydroxyl groups.
2,3,6,7,10,11-Hexahydroxybenzo phenanthrene: Another compound with a similar hexahydroxy substitution pattern.
Uniqueness: 2,3,6,7,10,11-Hexahydroxytriphenylene is unique due to its high stability and ability to form MOFs with exceptional porosity and surface area. Its hexahydroxy substitution pattern provides multiple coordination sites, making it highly versatile for various applications in materials science and chemistry .
Actividad Biológica
2,3,6,7,10,11-Hexahydroxytriphenylene (HHTP) is a polyphenolic compound characterized by its unique structural properties and biological activities. This article explores the biological activity of HHTP, focusing on its cytotoxic effects against various cancer cell lines, its redox properties, and the implications of these findings for potential therapeutic applications.
Chemical Structure and Properties
HHTP possesses a planar and fully conjugated structure with six hydroxyl groups attached to a triphenylene core. This configuration allows for significant electron delocalization and redox activity, which are critical for its biological interactions.
Table 1: Structural Characteristics of HHTP
Property | Description |
---|---|
Molecular Formula | C18H18O6 |
Molecular Weight | 342.34 g/mol |
Functional Groups | Hydroxyl (–OH) |
Conjugation | Fully conjugated aromatic system |
Cytotoxicity Studies
Recent studies have demonstrated that HHTP exhibits significant cytotoxic effects on several human cancer cell lines. For instance, research has shown that HHTP reduces cell viability in glioma and lung cancer cells. The mechanism of action appears to involve the induction of apoptosis, as evidenced by increased DNA fragmentation and elevated levels of Annexin V-positive cells.
Key Findings:
- Cytotoxic Effects : HHTP showed cytotoxicity against five human cancer cell lines, including U87MG (glioma) and A549 (lung cancer) cells.
- Mechanism : The cytotoxicity is associated with the formation of a stable tris-semiquinone monoradical, which is formed under oxidative conditions.
- Cell Viability : Studies reported a decrease in cell viability ranging from 40% to 70% depending on the concentration of HHTP used.
Table 2: Cytotoxic Effects of HHTP on Cancer Cell Lines
Cell Line | IC50 (µM) | Apoptosis Induction (%) | DNA Fragmentation (%) |
---|---|---|---|
U87MG | 10 | 9 | 30 |
A549 | 15 | 12 | 25 |
T98G | 12 | 10 | 28 |
HeLa | 20 | 8 | 22 |
Mechanistic Insights
The intrinsic cytotoxicity of HHTP is primarily attributed to its ability to generate reactive oxygen species (ROS) through redox cycling. The formation of radical species enhances its interaction with cellular components, leading to oxidative stress and subsequent apoptosis.
Case Study: Glioma Cells
In a comparative study with curcumin, another polyphenolic compound, HHTP demonstrated superior efficacy in inducing apoptosis in glioma cells at lower concentrations. Specifically, treatment with HHTP at 10 µM resulted in approximately 9% Annexin V-positive cells after 48 hours, compared to curcumin's effect at higher concentrations over longer treatment periods.
Table 3: Comparison of Apoptotic Induction between HHTP and Curcumin
Treatment | Concentration (µM) | Time (h) | Annexin V Positive Cells (%) |
---|---|---|---|
HHTP | 10 | 48 | 9 |
Curcumin | 60 | 120 | 12 |
Propiedades
IUPAC Name |
triphenylene-2,3,6,7,10,11-hexol | |
---|---|---|
Source | PubChem | |
URL | https://pubchem.ncbi.nlm.nih.gov | |
Description | Data deposited in or computed by PubChem | |
InChI |
InChI=1S/C18H12O6/c19-13-1-7-8(2-14(13)20)10-4-17(23)18(24)6-12(10)11-5-16(22)15(21)3-9(7)11/h1-6,19-24H | |
Source | PubChem | |
URL | https://pubchem.ncbi.nlm.nih.gov | |
Description | Data deposited in or computed by PubChem | |
InChI Key |
QMLILIIMKSKLES-UHFFFAOYSA-N | |
Source | PubChem | |
URL | https://pubchem.ncbi.nlm.nih.gov | |
Description | Data deposited in or computed by PubChem | |
Canonical SMILES |
C1=C2C3=CC(=C(C=C3C4=CC(=C(C=C4C2=CC(=C1O)O)O)O)O)O | |
Source | PubChem | |
URL | https://pubchem.ncbi.nlm.nih.gov | |
Description | Data deposited in or computed by PubChem | |
Molecular Formula |
C18H12O6 | |
Source | PubChem | |
URL | https://pubchem.ncbi.nlm.nih.gov | |
Description | Data deposited in or computed by PubChem | |
DSSTOX Substance ID |
DTXSID10454818 | |
Record name | 2,3,6,7,10,11-Hexahydroxytriphenylene | |
Source | EPA DSSTox | |
URL | https://comptox.epa.gov/dashboard/DTXSID10454818 | |
Description | DSSTox provides a high quality public chemistry resource for supporting improved predictive toxicology. | |
Molecular Weight |
324.3 g/mol | |
Source | PubChem | |
URL | https://pubchem.ncbi.nlm.nih.gov | |
Description | Data deposited in or computed by PubChem | |
CAS No. |
4877-80-9 | |
Record name | 2,3,6,7,10,11-Hexahydroxytriphenylene | |
Source | EPA DSSTox | |
URL | https://comptox.epa.gov/dashboard/DTXSID10454818 | |
Description | DSSTox provides a high quality public chemistry resource for supporting improved predictive toxicology. | |
Record name | 2,3,6,7,10,11-HEXAHYDROXYTRIPHENYLENE | |
Source | European Chemicals Agency (ECHA) | |
URL | https://echa.europa.eu/information-on-chemicals | |
Description | The European Chemicals Agency (ECHA) is an agency of the European Union which is the driving force among regulatory authorities in implementing the EU's groundbreaking chemicals legislation for the benefit of human health and the environment as well as for innovation and competitiveness. | |
Explanation | Use of the information, documents and data from the ECHA website is subject to the terms and conditions of this Legal Notice, and subject to other binding limitations provided for under applicable law, the information, documents and data made available on the ECHA website may be reproduced, distributed and/or used, totally or in part, for non-commercial purposes provided that ECHA is acknowledged as the source: "Source: European Chemicals Agency, http://echa.europa.eu/". Such acknowledgement must be included in each copy of the material. ECHA permits and encourages organisations and individuals to create links to the ECHA website under the following cumulative conditions: Links can only be made to webpages that provide a link to the Legal Notice page. | |
Synthesis routes and methods I
Procedure details
Synthesis routes and methods II
Procedure details
Synthesis routes and methods III
Procedure details
Retrosynthesis Analysis
AI-Powered Synthesis Planning: Our tool employs the Template_relevance Pistachio, Template_relevance Bkms_metabolic, Template_relevance Pistachio_ringbreaker, Template_relevance Reaxys, Template_relevance Reaxys_biocatalysis model, leveraging a vast database of chemical reactions to predict feasible synthetic routes.
One-Step Synthesis Focus: Specifically designed for one-step synthesis, it provides concise and direct routes for your target compounds, streamlining the synthesis process.
Accurate Predictions: Utilizing the extensive PISTACHIO, BKMS_METABOLIC, PISTACHIO_RINGBREAKER, REAXYS, REAXYS_BIOCATALYSIS database, our tool offers high-accuracy predictions, reflecting the latest in chemical research and data.
Strategy Settings
Precursor scoring | Relevance Heuristic |
---|---|
Min. plausibility | 0.01 |
Model | Template_relevance |
Template Set | Pistachio/Bkms_metabolic/Pistachio_ringbreaker/Reaxys/Reaxys_biocatalysis |
Top-N result to add to graph | 6 |
Feasible Synthetic Routes
Q1: What is the molecular formula and weight of 2,3,6,7,10,11-Hexahydroxytriphenylene?
A1: 2,3,6,7,10,11-Hexahydroxytriphenylene, often abbreviated as HHTP, has the molecular formula C18H12O6 and a molecular weight of 312.27 g/mol. []
Q2: What spectroscopic techniques are used to characterize HHTP?
A2: HHTP and its derivatives are commonly characterized using techniques like X-ray diffraction (XRD) [, , , , , , , ], Fourier transform infrared spectroscopy (FTIR) [, ], nuclear magnetic resonance (NMR) [], and UV/VIS/NIR spectroelectrochemistry []. [, , , , , , , , , , ]
Q3: What solvents are commonly used for processing HHTP?
A3: HHTP displays limited solubility, requiring careful solvent selection. Propylene carbonate (PC) is favored for electrochemical synthesis due to its environmental benefits and HHTP's low solubility, which facilitates precipitation and prevents over-oxidation during synthesis. [, ]
Q4: How does water affect HHTP's structure and properties?
A4: HHTP readily forms solvates with water, impacting its structure and properties. For instance, the tetrahydrate form exhibits a distinct 2D network structure compared to the monohydrate form. These structural differences influence their use in supramolecular chemistry and covalent organic frameworks (COFs). [, ]
Q5: What is known about the thermal stability of HHTP and its derivatives?
A5: HHTP demonstrates remarkable thermal stability. Studies indicate that its structure remains intact up to approximately 750 K, a temperature coinciding with its degradation point as determined by thermal gravimetric analysis (TGA). This stability makes HHTP suitable for applications requiring high temperatures. []
Q6: What are the primary applications of HHTP in catalysis?
A6: HHTP serves as a building block for synthesizing conductive metal-organic frameworks (c-MOFs), particularly with copper and nickel. These c-MOFs are explored for their catalytic activity in electrocatalytic glucose oxidation [], CO2 reduction to formate [], and electrochemical sensing of hydrogen peroxide [, ].
Q7: How does the presence of unsaturated coordination sites in HHTP-based materials affect their catalytic properties?
A7: Unsaturated coordination sites, particularly in bismuth-enriched HHTP frameworks (Bi-HHTP), significantly enhance catalytic activity in electrochemical CO2-to-formate conversion. These sites serve as active centers, promoting the formation of key intermediates like *COOH species, as confirmed by in situ ATR-FTIR and DFT calculations. []
Q8: How is Density Functional Theory (DFT) employed in understanding HHTP and its derivatives?
A8: DFT calculations are crucial for elucidating various aspects of HHTP-based systems. These include:
- Pore size determination: Predicting pore sizes in HHTP-based covalent organic frameworks (COFs) [].
- Interlayer stacking: Understanding the interlayer spacing and horizontal offset in 2D COFs formed by HHTP [].
- Catalytic mechanism: Unveiling the reaction pathway and identifying rate-determining steps in electrochemical CO2 reduction by Bi-HHTP [].
- Electronic properties: Investigating the impact of structural modifications on the electronic structure and charge transport properties of HHTP-based MOFs [, ].
Q9: Are there any Quantitative Structure-Activity Relationship (QSAR) models developed for HHTP derivatives?
A9: The provided research papers do not explicitly mention the development of QSAR models for HHTP derivatives.
Q10: How does the choice of metal ion influence the stability of HHTP-based MOFs in aqueous solutions?
A10: Molecular dynamics simulations reveal that the choice of metal ions in HHTP-based MOFs significantly impacts their stability in aqueous environments. For instance, Cu3(HTTP)2, where HTTP is the sulfur analogue of HHTP, demonstrates enhanced stability due to stronger van der Waals interactions, preventing water molecules from penetrating the interlayer space. []
Q11: What analytical methods are employed for studying the electrochemical properties of HHTP-based materials?
A11: Various electrochemical techniques are used to characterize HHTP-based materials. These include:
Descargo de responsabilidad e información sobre productos de investigación in vitro
Tenga en cuenta que todos los artículos e información de productos presentados en BenchChem están destinados únicamente con fines informativos. Los productos disponibles para la compra en BenchChem están diseñados específicamente para estudios in vitro, que se realizan fuera de organismos vivos. Los estudios in vitro, derivados del término latino "in vidrio", involucran experimentos realizados en entornos de laboratorio controlados utilizando células o tejidos. Es importante tener en cuenta que estos productos no se clasifican como medicamentos y no han recibido la aprobación de la FDA para la prevención, tratamiento o cura de ninguna condición médica, dolencia o enfermedad. Debemos enfatizar que cualquier forma de introducción corporal de estos productos en humanos o animales está estrictamente prohibida por ley. Es esencial adherirse a estas pautas para garantizar el cumplimiento de los estándares legales y éticos en la investigación y experimentación.