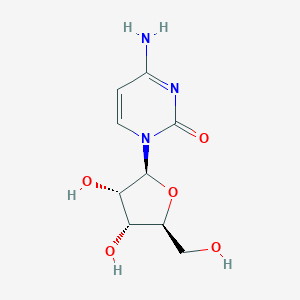
4-Amino-1-((2S,3S,4R,5S)-3,4-dihydroxy-5-(hydroxymethyl)tetrahydrofuran-2-yl)pyrimidin-2(1H)-one
Descripción general
Descripción
4-Amino-1-((2S,3S,4R,5S)-3,4-dihydroxy-5-(hydroxymethyl)tetrahydrofuran-2-yl)pyrimidin-2(1H)-one is a natural product found in Morinda citrifolia with data available.
Aplicaciones Científicas De Investigación
Antiviral Drug Synthesis
L-Cytidine is utilized in the synthesis of antiviral drugs, such as Molnupiravir, which is an orally available treatment for SARS-CoV-2 currently in phase 3 clinical trials. The biocatalytic process for Molnupiravir highlights the importance of cost-effective manufacturing of antiviral drugs at scale .
Metabolic Pathway Analysis
Metabolic control analysis (MCA) uses L-Cytidine to analyze metabolic pathways, particularly in the production processes of compounds like L-cysteine. This analysis helps in understanding and optimizing metabolic steady states and fluxome databases .
Enzymatic Assays
L-Cytidine plays a role in enzymatic assays, where it is used to determine concentrations of various compounds. For example, a new enzymatic assay for determining L-cysteine concentration involves the use of C-S lyase, which catalyzes the elimination of L-cysteine to produce measurable by-products .
Mecanismo De Acción
Target of Action
L-Cytidine primarily targets the enzyme Uridine-cytidine kinase 2 . This enzyme plays a crucial role in the pyrimidine salvage pathway, which is essential for nucleotide synthesis .
Mode of Action
As an analogue of cytidine, L-Cytidine can incorporate into RNA and DNA, disrupting RNA metabolism and inhibiting protein and DNA synthesis . It can also inhibit DNA methyltransferase, impairing DNA methylation .
Biochemical Pathways
L-Cytidine affects the pyrimidine salvage pathway, which is responsible for the recycling of pyrimidines to synthesize nucleotides . It also impacts the de novo pathway of nucleotide synthesis .
Pharmacokinetics
It is known that its absorption, distribution, metabolism, and excretion (adme) properties significantly impact its bioavailability .
Result of Action
The incorporation of L-Cytidine into RNA and DNA and its inhibition of DNA methylation can lead to changes in gene expression . This can have various molecular and cellular effects, including the management of neuropsychiatric deficits associated with cerebrovascular diseases when used in combination with uridine .
Action Environment
The action of L-Cytidine can be influenced by various environmental factors. For instance, adverse cellular conditions such as chronic inflammation can lead to its deregulation and overexpression . This can have significant implications for its action, efficacy, and stability.
Propiedades
IUPAC Name |
4-amino-1-[(2S,3S,4R,5S)-3,4-dihydroxy-5-(hydroxymethyl)oxolan-2-yl]pyrimidin-2-one | |
---|---|---|
Source | PubChem | |
URL | https://pubchem.ncbi.nlm.nih.gov | |
Description | Data deposited in or computed by PubChem | |
InChI |
InChI=1S/C9H13N3O5/c10-5-1-2-12(9(16)11-5)8-7(15)6(14)4(3-13)17-8/h1-2,4,6-8,13-15H,3H2,(H2,10,11,16)/t4-,6-,7-,8-/m0/s1 | |
Source | PubChem | |
URL | https://pubchem.ncbi.nlm.nih.gov | |
Description | Data deposited in or computed by PubChem | |
InChI Key |
UHDGCWIWMRVCDJ-PSQAKQOGSA-N | |
Source | PubChem | |
URL | https://pubchem.ncbi.nlm.nih.gov | |
Description | Data deposited in or computed by PubChem | |
Canonical SMILES |
C1=CN(C(=O)N=C1N)C2C(C(C(O2)CO)O)O | |
Source | PubChem | |
URL | https://pubchem.ncbi.nlm.nih.gov | |
Description | Data deposited in or computed by PubChem | |
Isomeric SMILES |
C1=CN(C(=O)N=C1N)[C@@H]2[C@H]([C@H]([C@@H](O2)CO)O)O | |
Source | PubChem | |
URL | https://pubchem.ncbi.nlm.nih.gov | |
Description | Data deposited in or computed by PubChem | |
Molecular Formula |
C9H13N3O5 | |
Source | PubChem | |
URL | https://pubchem.ncbi.nlm.nih.gov | |
Description | Data deposited in or computed by PubChem | |
DSSTOX Substance ID |
DTXSID40276262 | |
Record name | L-Cytidine | |
Source | EPA DSSTox | |
URL | https://comptox.epa.gov/dashboard/DTXSID40276262 | |
Description | DSSTox provides a high quality public chemistry resource for supporting improved predictive toxicology. | |
Molecular Weight |
243.22 g/mol | |
Source | PubChem | |
URL | https://pubchem.ncbi.nlm.nih.gov | |
Description | Data deposited in or computed by PubChem | |
Product Name |
4-Amino-1-((2S,3S,4R,5S)-3,4-dihydroxy-5-(hydroxymethyl)tetrahydrofuran-2-yl)pyrimidin-2(1H)-one | |
CAS RN |
26524-60-7 | |
Record name | L-Cytidine | |
Source | EPA DSSTox | |
URL | https://comptox.epa.gov/dashboard/DTXSID40276262 | |
Description | DSSTox provides a high quality public chemistry resource for supporting improved predictive toxicology. | |
Retrosynthesis Analysis
AI-Powered Synthesis Planning: Our tool employs the Template_relevance Pistachio, Template_relevance Bkms_metabolic, Template_relevance Pistachio_ringbreaker, Template_relevance Reaxys, Template_relevance Reaxys_biocatalysis model, leveraging a vast database of chemical reactions to predict feasible synthetic routes.
One-Step Synthesis Focus: Specifically designed for one-step synthesis, it provides concise and direct routes for your target compounds, streamlining the synthesis process.
Accurate Predictions: Utilizing the extensive PISTACHIO, BKMS_METABOLIC, PISTACHIO_RINGBREAKER, REAXYS, REAXYS_BIOCATALYSIS database, our tool offers high-accuracy predictions, reflecting the latest in chemical research and data.
Strategy Settings
Precursor scoring | Relevance Heuristic |
---|---|
Min. plausibility | 0.01 |
Model | Template_relevance |
Template Set | Pistachio/Bkms_metabolic/Pistachio_ringbreaker/Reaxys/Reaxys_biocatalysis |
Top-N result to add to graph | 6 |
Feasible Synthetic Routes
Q & A
Q1: What makes L-Cytidine a potential antiviral agent?
A1: Unlike its naturally occurring D-enantiomer, L-Cytidine demonstrates potent antiviral activity against human immunodeficiency virus type 1 (HIV-1) and hepatitis B virus (HBV) in vitro. [, , ] This is attributed to its unique interaction with viral enzymes, inhibiting their replication cycle. [, ]
Q2: How does L-Cytidine compare to its D-enantiomer (D-Cytidine) in terms of antiviral activity?
A2: L-Cytidine exhibits significantly higher potency against HBV compared to D-Cytidine. Specifically, L-Cytidine is approximately 1000 times more potent than its D-counterpart, 2',3'-dideoxy-beta-D-5-fluorocytidine (beta-D-FddC). [] This difference highlights the importance of stereochemistry in antiviral drug design.
Q3: How does L-Cytidine exert its antiviral effects against HIV-1 and HBV?
A3: While the precise mechanism of action is still under investigation, L-Cytidine likely acts as a chain terminator during viral DNA synthesis. [, ] It's suggested that L-Cytidine is phosphorylated within the cell to its active triphosphate form, which then competes with natural nucleotides for incorporation into the growing viral DNA chain, ultimately halting further elongation.
Q4: Does L-Cytidine show any activity against other viruses?
A4: Research has shown that L-Cytidine and its 5-fluoro derivative, 2′,3′-Dideoxy-β-L-5-fluorocytidine (β-L-FddC), do not exhibit activity against Herpes Simplex Viruses 1 and 2. [] This suggests a degree of specificity towards HIV-1 and HBV.
Q5: Are there any known toxicities associated with L-Cytidine?
A5: While L-Cytidine shows a favorable toxicity profile compared to ddC, it has exhibited in vitro cellular toxicity in several leukocytic cell lines. [] Additionally, it has been shown to inhibit phytohemagglutinin-stimulated human peripheral blood mononuclear leukocyte proliferation, suggesting potential immunomodulatory effects. [, ] Further research is needed to fully elucidate its safety profile in vivo.
Q6: Has L-Cytidine been tested in clinical trials?
A6: To date, there are no published reports of clinical trials involving L-Cytidine. Further preclinical studies are crucial to assess its efficacy and safety before advancing to human trials.
Q7: What is the molecular formula and weight of L-Cytidine?
A7: The molecular formula of L-Cytidine is C9H13N3O5, and its molecular weight is 243.22 g/mol.
Q8: What is the impact of structural modifications on the activity of L-Cytidine?
A8: Research suggests that even minor modifications to the L-Cytidine structure can significantly impact its antiviral activity and cytotoxicity. For instance, the addition of a fluorine atom at the 5-position of the cytosine base, creating β-L-FddC, leads to enhanced anti-HIV activity compared to the unmodified L-Cytidine. [, ] Conversely, the 4'-thio-L-nucleosides, incorporating a sulfur atom in the ribose ring, did not exhibit antiviral activity against HIV or HBV. [] This highlights the importance of exploring structure-activity relationships (SAR) to optimize the antiviral potency and safety of L-Cytidine derivatives.
Q9: Have any prodrugs of L-Cytidine been developed?
A9: Yes, to enhance the oral bioavailability of L-Cytidine, researchers have synthesized various prodrugs, such as the 3′-mono-, 5′-mono-, and 3′,5′-di-O-valinyl esters. [] Among these, the 3′-mono-O-valinyl derivative has shown promise as a potential anti-HBV agent due to its favorable pharmacokinetic profile in cynomolgus monkeys. []
Descargo de responsabilidad e información sobre productos de investigación in vitro
Tenga en cuenta que todos los artículos e información de productos presentados en BenchChem están destinados únicamente con fines informativos. Los productos disponibles para la compra en BenchChem están diseñados específicamente para estudios in vitro, que se realizan fuera de organismos vivos. Los estudios in vitro, derivados del término latino "in vidrio", involucran experimentos realizados en entornos de laboratorio controlados utilizando células o tejidos. Es importante tener en cuenta que estos productos no se clasifican como medicamentos y no han recibido la aprobación de la FDA para la prevención, tratamiento o cura de ninguna condición médica, dolencia o enfermedad. Debemos enfatizar que cualquier forma de introducción corporal de estos productos en humanos o animales está estrictamente prohibida por ley. Es esencial adherirse a estas pautas para garantizar el cumplimiento de los estándares legales y éticos en la investigación y experimentación.