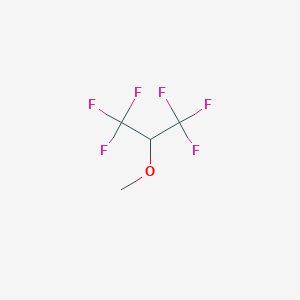
Hexafluoroisopropyl methyl ether
Descripción general
Descripción
Hexafluoroisopropyl methyl ether (HFPM) is a fluorinated ether, also known as isoflurothyl . It is a colorless, non-flammable liquid used in the battery industry as a high-performing cosolvent and additive for electrolyte formulations in lithium-ion batteries .
Synthesis Analysis
Hexafluoroisopropyl methyl ether can be synthesized using hexafluoroisopropanol and methyl chloride . Another method involves adding 185g of methyl chloride, 300g of sodium carbonate, and 3.5L of dimethyl sulfoxide to a reactor. After stirring at room temperature for three hours, 168g of hexafluoroisopropanol is added. The temperature is controlled at 80-90°C, and the reaction is stirred for 12 hours .Molecular Structure Analysis
The molecular formula of Hexafluoroisopropyl methyl ether is C4H4F6O . The molecule contains a total of 14 bonds. There are 10 non-H bonds, 1 rotatable bond, and 1 ether (aliphatic) .Chemical Reactions Analysis
Hexafluoroisopropyl methyl ether has been found to be involved in the hydroarylation of enamides . It forms rapidly and serves as a slow-release reservoir for the key cationic intermediate, preventing the oligomerization of the substrate under the reaction conditions .Physical And Chemical Properties Analysis
Hexafluoroisopropyl methyl ether has a molecular weight of 182.07 . It is a liquid at 20°C . The boiling point is 50°C , and the specific gravity is 1.39 . The refractive index is 1.28 .Aplicaciones Científicas De Investigación
Battery Manufacturing
HFPM is used in the battery industry as a high-performing cosolvent and additive for electrolyte formulations in lithium-ion batteries . It has a low viscosity and a low boiling point and is miscible with many polar organic solvents, which lend it use as a diluent for low-temperature applications .
Safety in Battery Applications
The nonflammability of HFPM is a major benefit for battery safety . It also has good compatibility with most electrode materials including, for example, lithium metal, graphite, NMCs, and LMO .
Sodium-ion Batteries (SIBs)
In SIBs, adding HFPM as an electrolyte additive can help to stabilize the Na electrode surface and form a new fluorine-containing organic layer, resulting in a more stable solid electrolyte interphase .
Biomedical Applications
HFPM, also called isoflurothyl, is used in biomedical applications as a general anesthetic .
Hydroarylation of Enamides
HFPM greatly expands the scope with respect to both reaction partners of the Brønsted acid-catalyzed hydroarylation of enamides . The reaction is fast and practical and can be performed on the gram scale .
Synthesis of Hexafluoroisopropyl Ethers
HFPM can be synthesized in a single step reaction involving the compound methoxymalononitrile and a bromine trifluoride composition .
Mecanismo De Acción
Target of Action
Hexafluoroisopropyl methyl ether (HFPM) is a fluorinated ether . It is primarily used as a high-performing cosolvent and additive for electrolyte formulations in lithium-ion batteries (LiBs) and sodium-ion batteries (SIBs) . Therefore, its primary targets are the components of these batteries, including the electrodes and the electrolyte solution .
Mode of Action
HFPM interacts with its targets by serving as a cosolvent in the electrolyte solution of LiBs and SIBs . It has a low viscosity and a low boiling point, and is miscible with many polar organic solvents . This makes it an ideal diluent for low-temperature applications and can help to improve the wettability of polypropylene separators .
Biochemical Pathways
In the context of battery technology, HFPM affects the electrochemical pathways involved in the operation of LiBs and SIBs . Studies have shown that adding HFPM as an electrolyte additive can help to stabilize the Na electrode surface and form a new fluorine-containing organic layer . This results in a more stable solid electrolyte interphase .
Pharmacokinetics
While the term “pharmacokinetics” is typically used in the context of drug metabolism, in the case of HFPM, we can discuss its properties that affect its behavior in the battery system. HFPM has a low viscosity and a low boiling point . These properties influence its distribution within the battery system and its interaction with other components.
Result of Action
The primary result of HFPM’s action is the stabilization of the electrode surface in SIBs . This leads to the formation of a more stable solid electrolyte interphase . Additionally, HFPM’s nonflammability is a major benefit for battery safety . It also has good compatibility with most electrode materials .
Action Environment
The action of HFPM can be influenced by various environmental factors. For instance, its effectiveness as a cosolvent can be affected by temperature, given its low boiling point . Furthermore, its nonflammability makes it a safer choice in environments where fire safety is a concern .
Direcciones Futuras
Hexafluoroisopropyl methyl ether has shown potential in the battery industry, particularly in the development of lithium-ion batteries . Its low viscosity, low boiling point, and compatibility with a variety of battery solvents make it an ideal diluent for low-temperature applications . It also has good compatibility with most electrode materials .
Propiedades
IUPAC Name |
1,1,1,3,3,3-hexafluoro-2-methoxypropane | |
---|---|---|
Source | PubChem | |
URL | https://pubchem.ncbi.nlm.nih.gov | |
Description | Data deposited in or computed by PubChem | |
InChI |
InChI=1S/C4H4F6O/c1-11-2(3(5,6)7)4(8,9)10/h2H,1H3 | |
Source | PubChem | |
URL | https://pubchem.ncbi.nlm.nih.gov | |
Description | Data deposited in or computed by PubChem | |
InChI Key |
VNXYDFNVQBICRO-UHFFFAOYSA-N | |
Source | PubChem | |
URL | https://pubchem.ncbi.nlm.nih.gov | |
Description | Data deposited in or computed by PubChem | |
Canonical SMILES |
COC(C(F)(F)F)C(F)(F)F | |
Source | PubChem | |
URL | https://pubchem.ncbi.nlm.nih.gov | |
Description | Data deposited in or computed by PubChem | |
Molecular Formula |
C4H4F6O | |
Source | PubChem | |
URL | https://pubchem.ncbi.nlm.nih.gov | |
Description | Data deposited in or computed by PubChem | |
DSSTOX Substance ID |
DTXSID80157187 | |
Record name | Hexafluoroisopropyl methyl ether | |
Source | EPA DSSTox | |
URL | https://comptox.epa.gov/dashboard/DTXSID80157187 | |
Description | DSSTox provides a high quality public chemistry resource for supporting improved predictive toxicology. | |
Molecular Weight |
182.06 g/mol | |
Source | PubChem | |
URL | https://pubchem.ncbi.nlm.nih.gov | |
Description | Data deposited in or computed by PubChem | |
Product Name |
Hexafluoroisopropyl methyl ether | |
CAS RN |
13171-18-1 | |
Record name | Hexafluoroisopropyl methyl ether | |
Source | CAS Common Chemistry | |
URL | https://commonchemistry.cas.org/detail?cas_rn=13171-18-1 | |
Description | CAS Common Chemistry is an open community resource for accessing chemical information. Nearly 500,000 chemical substances from CAS REGISTRY cover areas of community interest, including common and frequently regulated chemicals, and those relevant to high school and undergraduate chemistry classes. This chemical information, curated by our expert scientists, is provided in alignment with our mission as a division of the American Chemical Society. | |
Explanation | The data from CAS Common Chemistry is provided under a CC-BY-NC 4.0 license, unless otherwise stated. | |
Record name | Iso-indoklon | |
Source | ChemIDplus | |
URL | https://pubchem.ncbi.nlm.nih.gov/substance/?source=chemidplus&sourceid=0013171181 | |
Description | ChemIDplus is a free, web search system that provides access to the structure and nomenclature authority files used for the identification of chemical substances cited in National Library of Medicine (NLM) databases, including the TOXNET system. | |
Record name | Propane, 1,1,1,3,3,3-hexafluoro-2-methoxy- | |
Source | EPA Chemicals under the TSCA | |
URL | https://www.epa.gov/chemicals-under-tsca | |
Description | EPA Chemicals under the Toxic Substances Control Act (TSCA) collection contains information on chemicals and their regulations under TSCA, including non-confidential content from the TSCA Chemical Substance Inventory and Chemical Data Reporting. | |
Record name | Hexafluoroisopropyl methyl ether | |
Source | EPA DSSTox | |
URL | https://comptox.epa.gov/dashboard/DTXSID80157187 | |
Description | DSSTox provides a high quality public chemistry resource for supporting improved predictive toxicology. | |
Record name | 1,1,1,3,3,3-hexafluoro-2-methoxypropane | |
Source | European Chemicals Agency (ECHA) | |
URL | https://echa.europa.eu/substance-information/-/substanceinfo/100.124.805 | |
Description | The European Chemicals Agency (ECHA) is an agency of the European Union which is the driving force among regulatory authorities in implementing the EU's groundbreaking chemicals legislation for the benefit of human health and the environment as well as for innovation and competitiveness. | |
Explanation | Use of the information, documents and data from the ECHA website is subject to the terms and conditions of this Legal Notice, and subject to other binding limitations provided for under applicable law, the information, documents and data made available on the ECHA website may be reproduced, distributed and/or used, totally or in part, for non-commercial purposes provided that ECHA is acknowledged as the source: "Source: European Chemicals Agency, http://echa.europa.eu/". Such acknowledgement must be included in each copy of the material. ECHA permits and encourages organisations and individuals to create links to the ECHA website under the following cumulative conditions: Links can only be made to webpages that provide a link to the Legal Notice page. | |
Record name | 1,1,1,3,3,3-HEXAFLUORO-2-METHOXY-PROPANE | |
Source | FDA Global Substance Registration System (GSRS) | |
URL | https://gsrs.ncats.nih.gov/ginas/app/beta/substances/N8YMW3PAU7 | |
Description | The FDA Global Substance Registration System (GSRS) enables the efficient and accurate exchange of information on what substances are in regulated products. Instead of relying on names, which vary across regulatory domains, countries, and regions, the GSRS knowledge base makes it possible for substances to be defined by standardized, scientific descriptions. | |
Explanation | Unless otherwise noted, the contents of the FDA website (www.fda.gov), both text and graphics, are not copyrighted. They are in the public domain and may be republished, reprinted and otherwise used freely by anyone without the need to obtain permission from FDA. Credit to the U.S. Food and Drug Administration as the source is appreciated but not required. | |
Synthesis routes and methods
Procedure details
Retrosynthesis Analysis
AI-Powered Synthesis Planning: Our tool employs the Template_relevance Pistachio, Template_relevance Bkms_metabolic, Template_relevance Pistachio_ringbreaker, Template_relevance Reaxys, Template_relevance Reaxys_biocatalysis model, leveraging a vast database of chemical reactions to predict feasible synthetic routes.
One-Step Synthesis Focus: Specifically designed for one-step synthesis, it provides concise and direct routes for your target compounds, streamlining the synthesis process.
Accurate Predictions: Utilizing the extensive PISTACHIO, BKMS_METABOLIC, PISTACHIO_RINGBREAKER, REAXYS, REAXYS_BIOCATALYSIS database, our tool offers high-accuracy predictions, reflecting the latest in chemical research and data.
Strategy Settings
Precursor scoring | Relevance Heuristic |
---|---|
Min. plausibility | 0.01 |
Model | Template_relevance |
Template Set | Pistachio/Bkms_metabolic/Pistachio_ringbreaker/Reaxys/Reaxys_biocatalysis |
Top-N result to add to graph | 6 |
Feasible Synthetic Routes
Q & A
Q1: What are the primary applications of 1,1,1,3,3,3-Hexafluoroisopropyl methyl ether?
A: 1,1,1,3,3,3-Hexafluoroisopropyl methyl ether (HFPM) demonstrates potential as a component in electrolytes for lithium-ion batteries [, ]. Its electrochemical properties contribute to enhanced safety and longevity in these applications. Additionally, HFPM has been studied for its anesthetic properties in vivo [].
Q2: Are there environmental concerns associated with 1,1,1,3,3,3-Hexafluoroisopropyl methyl ether?
A: While the provided research focuses primarily on synthesis and applications of HFPM, one study estimated its atmospheric lifetime to be 67 days []. This suggests a potential for environmental persistence. Further research is needed to fully assess its ecotoxicological effects and degradation pathways.
Q3: What is the molecular formula and weight of 1,1,1,3,3,3-Hexafluoroisopropyl methyl ether?
A: The molecular formula of HFPM is C4H6F6O, and its molecular weight is 184.09 g/mol [].
Q4: What synthetic routes are available for producing 1,1,1,3,3,3-Hexafluoroisopropyl methyl ether?
A: Several methods for synthesizing HFPM exist, including a catalytic method using hexafluoroisopropanol and iodomethane as starting materials []. Another approach utilizes hexafluoroisopropanol, methyl carbonate, and an alkali catalyst at elevated temperatures [].
Q5: How does the structure of 1,1,1,3,3,3-Hexafluoroisopropyl methyl ether influence its properties?
A: The presence of fluorine atoms in HFPM contributes to its low flammability and high electrochemical stability []. These properties are crucial for its potential use in lithium-ion batteries. Additionally, its structure influences its anesthetic activity, although this relationship is complex and not fully understood [].
Q6: Has research explored optimizing the synthesis of 1,1,1,3,3,3-Hexafluoroisopropyl methyl ether?
A: Yes, researchers have employed response surface analysis, specifically the Plackett-Burman and Box-Behnken designs, to optimize the synthesis process for HFPM []. This approach identified key factors influencing yield and purity, leading to improvements in production efficiency.
Q7: What analytical techniques are commonly used to study 1,1,1,3,3,3-Hexafluoroisopropyl methyl ether?
A: Researchers have employed UV and IR spectroscopy to characterize HFPM and determine its absorption characteristics []. Further analytical methods are likely employed for quality control and assurance during synthesis and application, but details are not specified in the provided abstracts.
Q8: Are there alternative compounds with similar properties to 1,1,1,3,3,3-Hexafluoroisopropyl methyl ether?
A: One study investigated 1,1,2,2-tetrafluoroethyl-2,2,3,3-tetrafluoropropyl ether (TTE) as a potential alternative to HFPM for use in lithium-ion batteries []. Both compounds are fluorinated ethers, but their specific properties and performance characteristics may differ.
Descargo de responsabilidad e información sobre productos de investigación in vitro
Tenga en cuenta que todos los artículos e información de productos presentados en BenchChem están destinados únicamente con fines informativos. Los productos disponibles para la compra en BenchChem están diseñados específicamente para estudios in vitro, que se realizan fuera de organismos vivos. Los estudios in vitro, derivados del término latino "in vidrio", involucran experimentos realizados en entornos de laboratorio controlados utilizando células o tejidos. Es importante tener en cuenta que estos productos no se clasifican como medicamentos y no han recibido la aprobación de la FDA para la prevención, tratamiento o cura de ninguna condición médica, dolencia o enfermedad. Debemos enfatizar que cualquier forma de introducción corporal de estos productos en humanos o animales está estrictamente prohibida por ley. Es esencial adherirse a estas pautas para garantizar el cumplimiento de los estándares legales y éticos en la investigación y experimentación.