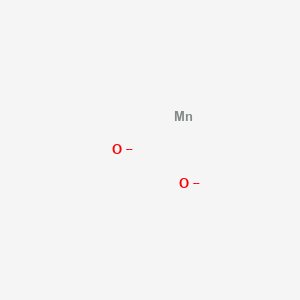
manganese(IV)dioxide
Descripción general
Descripción
Manganese(IV) dioxide (MnO2) is a highly reactive compound that plays a significant role in various environmental and chemical processes. It is known for its ability to oxidize a wide range of organic contaminants and its involvement in biogeochemical cycles . MnO2 is also utilized in oxidation reactions, such as the activation of strong C-H bonds in alkanes, which is not typically achieved via an oxygen-rebound mechanism .
Synthesis Analysis
The synthesis of MnO2 and its complexes can be achieved through different methods. For instance, a mononuclear non-heme manganese(IV)-oxo complex was synthesized using various spectroscopic methods . Another example is the synthesis of a novel manganese(IV) monomer, Mn(IV)(Me3TACN)(OMe)3, which was prepared by reacting MnCl2 with a specific ligand in the presence of Na2O2 . Additionally, manganese(IV) complexes have been synthesized by the oxidation of binuclear manganese(II) complexes with tetrachloro-o-benzoquinone .
Molecular Structure Analysis
The molecular structure of MnO2-based compounds can be quite complex. For example, the manganese(IV) monomer Mn(IV)(Me3TACN)(OMe)3 has a six-coordinate N3O3 ligand environment with specific bond distances and angles, indicating a facial coordination by the triazacyclononane . The structure of manganese(IV) complexes synthesized from manganese(II) complexes also shows magnetic moments corresponding to three unpaired electrons and strong absorption due to charge transfer transitions .
Chemical Reactions Analysis
Manganese(IV) dioxide is involved in various chemical reactions, including the reductive dissolution of manganese oxides by substituted phenols, which is important for the degradation of phenolic pollutants . It also promotes direct dehydrogenative alkylation of sp3 C-H bonds adjacent to a heteroatom . Furthermore, MnO2 can oxidize antimony(III) to antimony(V), enhancing its removal through an oxidation-adsorption mechanism .
Physical and Chemical Properties Analysis
The physical and chemical properties of MnO2 are influenced by its oxidation state, redox potential, and specific surface area. These properties are critical for its reactivity with organic contaminants and its potential use in water treatment applications . The diversity of manganese oxides at the nanoscale, including their structural, optical, catalytic, magnetic, and transport properties, opens up opportunities for applications in health and other fields . Additionally, the electrodeposition of MnO2 can result in preferred crystal growth, which is evident in the fibrous structure and marked texture of growth observed in certain forms of MnO2 .
Aplicaciones Científicas De Investigación
1. Industrial and Environmental Applications
Manganese(IV) dioxide (MnO2) is significant in industrial applications, particularly in steel production, catalysis, and battery manufacturing. MnO2, commonly found in manganese ores, is stable in acidic or alkaline oxidizing conditions. Its extraction is usually conducted under reducing conditions to convert insoluble Mn(IV) to soluble Mn(II) in aqueous media. This process involves either reduction roasting followed by leaching or a more energy-conserving method of hydrometallurgical reductive leaching, which may use various reducing agents, including agricultural and biomass wastes (Sinha & Purcell, 2019).
2. Biomedical Applications
Manganese dioxide nanomaterials have emerged as potential candidates for biomedical applications due to their simplicity in preparation, cost-effectiveness, and eco-friendliness. In tumor therapy, MnO2 nanomaterials primarily serve as drug carriers. They also play a role in alleviating tumor hypoxia by interacting with reactive oxygen species in the tumor microenvironment. Additionally, MnO2 nanosheets are utilized in constructing biosensors, gene therapy, and nuclear magnetic imaging (Chen, Cong, Shen, & Yu, 2020).
3. Chemical Synthesis
Manganese(IV) dioxide is used in chemical processes like the conversion of activated alcohols into esters and amides. This conversion can be achieved using MnO2 in conjunction with sodium cyanide in various solvents, facilitating the preparation of various organic compounds (Foot, Kanno, Giblin, & Taylor, 2003).
4. Environmental Purification
MnO2 is recognized for its potential in environmental purification, particularly in adsorbing heavy metals and degrading pollutants in water and gas. The efficiency of MnO2 in environmental applications has been enhanced through morphology control, structure construction, facet engineering, and element doping. Its applications extend to thermal catalysis, photocatalysis, and electrocatalysis for pollutant degradation (Yang et al., 2021).
5. Advanced Battery Applications
Manganese dioxide's polymorphic forms, controllable structure, and high porosity make it a valuable material in advanced batteries. The relationship between MnO2's structures and its electrochemical properties has been extensively studied, emphasizing its profound impact on battery performance (Yijian et al., 2018).
Safety And Hazards
Direcciones Futuras
MnO2 is considered as an exceptionally promising candidate catalyst for AOPs, owing to its low cost, high abundance, and environmental friendliness . The development of advanced cathode materials for zinc-ion batteries (ZIBs) is a critical step in building large-scale green energy conversion and storage systems in the future .
Propiedades
IUPAC Name |
manganese;oxygen(2-) | |
---|---|---|
Source | PubChem | |
URL | https://pubchem.ncbi.nlm.nih.gov | |
Description | Data deposited in or computed by PubChem | |
InChI |
InChI=1S/Mn.2O/q;2*-2 | |
Source | PubChem | |
URL | https://pubchem.ncbi.nlm.nih.gov | |
Description | Data deposited in or computed by PubChem | |
InChI Key |
WBJBOSCGOGORLE-UHFFFAOYSA-N | |
Source | PubChem | |
URL | https://pubchem.ncbi.nlm.nih.gov | |
Description | Data deposited in or computed by PubChem | |
Canonical SMILES |
[O-2].[O-2].[Mn] | |
Source | PubChem | |
URL | https://pubchem.ncbi.nlm.nih.gov | |
Description | Data deposited in or computed by PubChem | |
Molecular Formula |
MnO2-4 | |
Source | PubChem | |
URL | https://pubchem.ncbi.nlm.nih.gov | |
Description | Data deposited in or computed by PubChem | |
DSSTOX Substance ID |
DTXSID6042109 | |
Record name | Manganese dioxide | |
Source | EPA DSSTox | |
URL | https://comptox.epa.gov/dashboard/DTXSID6042109 | |
Description | DSSTox provides a high quality public chemistry resource for supporting improved predictive toxicology. | |
Molecular Weight |
86.937 g/mol | |
Source | PubChem | |
URL | https://pubchem.ncbi.nlm.nih.gov | |
Description | Data deposited in or computed by PubChem | |
Physical Description |
Dry Powder; Dry Powder, Pellets or Large Crystals; Other Solid; Pellets or Large Crystals, Other Solid; Water or Solvent Wet Solid | |
Record name | Manganese oxide (MnO2) | |
Source | EPA Chemicals under the TSCA | |
URL | https://www.epa.gov/chemicals-under-tsca | |
Description | EPA Chemicals under the Toxic Substances Control Act (TSCA) collection contains information on chemicals and their regulations under TSCA, including non-confidential content from the TSCA Chemical Substance Inventory and Chemical Data Reporting. | |
Product Name |
Manganese;oxygen(2-) | |
CAS RN |
1313-13-9 | |
Record name | Manganese oxide (MnO2) | |
Source | EPA Chemicals under the TSCA | |
URL | https://www.epa.gov/chemicals-under-tsca | |
Description | EPA Chemicals under the Toxic Substances Control Act (TSCA) collection contains information on chemicals and their regulations under TSCA, including non-confidential content from the TSCA Chemical Substance Inventory and Chemical Data Reporting. | |
Record name | Manganese dioxide | |
Source | EPA DSSTox | |
URL | https://comptox.epa.gov/dashboard/DTXSID6042109 | |
Description | DSSTox provides a high quality public chemistry resource for supporting improved predictive toxicology. | |
Record name | MANGANESE DIOXIDE | |
Source | FDA Global Substance Registration System (GSRS) | |
URL | https://gsrs.ncats.nih.gov/ginas/app/beta/substances/TF219GU161 | |
Description | The FDA Global Substance Registration System (GSRS) enables the efficient and accurate exchange of information on what substances are in regulated products. Instead of relying on names, which vary across regulatory domains, countries, and regions, the GSRS knowledge base makes it possible for substances to be defined by standardized, scientific descriptions. | |
Explanation | Unless otherwise noted, the contents of the FDA website (www.fda.gov), both text and graphics, are not copyrighted. They are in the public domain and may be republished, reprinted and otherwise used freely by anyone without the need to obtain permission from FDA. Credit to the U.S. Food and Drug Administration as the source is appreciated but not required. | |
Q & A
A: MnO2 exhibits its activity through redox reactions. For instance, it oxidizes various organic compounds, such as 2,3-naphthalenediol, leading to the reductive dissolution of MnO2 and the formation of oxidized organic products, including potentially insoluble polymers. [, ] In another example, MnO2 is reduced to Mn2+ by ascorbic acid, which is generated by the enzymatic activity of alkaline phosphatase on 2-phospho-L-ascorbic acid trisodium salt. This reduction reaction forms the basis of an ALP detection assay. []
A: MnO2 has a molecular formula of MnO2 and a molecular weight of 86.94 g/mol. While specific spectroscopic data depends on the polymorph and synthesis method, techniques like XRD, SEM, and EDX are commonly used for characterization. [, , ]
A: MnO2’s stability and activity are influenced by pH. In the oxidation of 2,3-naphthalenediol, the rate of reductive dissolution of MnO2 decreases with increasing pH, indicating proton concentration affects its reactivity. [, ]
A: MnO2 acts as a catalyst in various organic reactions. It enables the synthesis of quinoxalines from alpha-hydroxyketones and 1,2-diamines under microwave irradiation. [, , ] Additionally, it catalyzes the conversion of activated alcohols into methyl esters in conjunction with sodium cyanide. [] In environmental applications, MnO2 is used for removing sulfite from water through oxidation. []
A: Computer quantum-chemical simulations are employed to study the formation and properties of multicomponent systems involving MnO2. For example, simulations have shown the feasibility of creating SiO-MnO systems by adsorbing MnO2 nanoparticles onto SiO microspheres. []
A: Doping MnO2 with transition metal cations like Co3+ and Fe3+ modifies its ion exchange properties. This doping enhances the affinity for heavy alkaline earth ions (Ba2+ and Ra2+) compared to pure MnO2. []
A: While the provided research doesn’t directly address MnO2 formulation, its stability is influenced by factors like pH and the presence of reducing agents. [, ]
A: While MnO2 itself is naturally occurring and generally considered environmentally benign, the synthesis and some applications might have environmental implications. Research on its use for removing sulfite from water using a MnO2 microcolumn highlights its potential in environmental remediation. [] Specific alternatives depend on the application, with activated carbon being one option for oxidation reactions. []
Descargo de responsabilidad e información sobre productos de investigación in vitro
Tenga en cuenta que todos los artículos e información de productos presentados en BenchChem están destinados únicamente con fines informativos. Los productos disponibles para la compra en BenchChem están diseñados específicamente para estudios in vitro, que se realizan fuera de organismos vivos. Los estudios in vitro, derivados del término latino "in vidrio", involucran experimentos realizados en entornos de laboratorio controlados utilizando células o tejidos. Es importante tener en cuenta que estos productos no se clasifican como medicamentos y no han recibido la aprobación de la FDA para la prevención, tratamiento o cura de ninguna condición médica, dolencia o enfermedad. Debemos enfatizar que cualquier forma de introducción corporal de estos productos en humanos o animales está estrictamente prohibida por ley. Es esencial adherirse a estas pautas para garantizar el cumplimiento de los estándares legales y éticos en la investigación y experimentación.