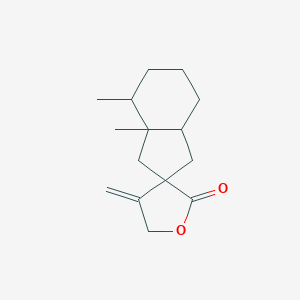
Bakkenolide A
Descripción general
Descripción
Aplicaciones Científicas De Investigación
Bakkenolide A has several scientific research applications:
Chemistry: It serves as a model compound for studying sesquiterpene lactones and their synthetic routes.
Medicine: Research is ongoing to explore its potential as an anticancer agent due to its cytotoxic properties.
Industry: Its insect antifeedant properties make it a candidate for developing natural insect repellents.
Mecanismo De Acción
Target of Action
Bakkenolide A, a natural product extracted from Petasites tricholobus, primarily targets Histone Deacetylase 3 (HDAC3) and the PI3K/Akt signaling pathway . HDAC3 plays a vital role in cancer formation and persistence through cell death, apoptosis, and inflammation .
Mode of Action
This compound interacts with its targets by regulating the activity of HDAC3 and the PI3K/Akt-related signaling pathways . This regulation results in changes in cell death, apoptosis, and inflammation, which are crucial processes in cancer formation and persistence .
Biochemical Pathways
The primary biochemical pathways affected by this compound are those related to HDAC3 and PI3K/Akt . The compound’s interaction with these targets leads to downstream effects such as the inhibition of inflammation, induction of apoptosis and cell death, down-regulation of IKKs expression, and suppression of proinflammatory cytokines of IL-1β, IL-18, and TNF-α .
Pharmacokinetics
A study on rats showed that after a single oral dose of 20 mg/kg of this compound, the mean peak plasma concentration (Cmax) of this compound was 234.7 ± 161 ng/mL at 0.25 h . The area under the plasma concentration-time curve (AUC0-24h) was 535.8 ± 223.7 h·ng/mL, and the elimination half-life (T1/2) was 5.0 ± 0.36 h . In case of intravenous administration of this compound at a dosage of 2 mg/kg, the AUC0-24h was 342 ± 98 h⋅ng/mL, and the T1/2 was 5.8 ± 0.7 h . Based on these results, the oral bioavailability of this compound in rats at 20 mg/kg is 15.7% .
Result of Action
The molecular and cellular effects of this compound’s action include the inhibition of inflammation, induction of apoptosis and cell death, down-regulation of IKKs expression, and suppression of proinflammatory cytokines of IL-1β, IL-18, and TNF-α . In HDAC3-knockdown and this compound-treated cells, an upregulation of Caspase3/7 was observed, inducing apoptosis in leukemia cells .
Análisis Bioquímico
Biochemical Properties
Bakkenolide A has been found to inhibit bacterial neuraminidase (NA), an enzyme that plays a crucial role in the life cycle of certain bacteria . The inhibition of NA by this compound suggests that it may interact with this enzyme, potentially binding to its active site and preventing it from carrying out its normal function .
Cellular Effects
The inhibition of NA by this compound can have significant effects on cellular processes. By preventing NA from functioning properly, this compound may disrupt the life cycle of bacteria that rely on this enzyme, potentially leading to their death .
Molecular Mechanism
It is believed that this compound may bind to the active site of NA, preventing it from interacting with its normal substrates . This could result in the inhibition of NA activity, disrupting the life cycle of bacteria that rely on this enzyme .
Métodos De Preparación
Synthetic Routes and Reaction Conditions: The total synthesis of Bakkenolide A involves several key steps. One notable method includes the asymmetric cyclization-carbonylation reaction, which is catalyzed by palladium(II) with chiral bisoxazoline ligands . The diastereoselective construction of the spirolactone moiety is achieved using Mitsuhashi’s protocol . The synthesis begins with the preparation of optically active hydrindanone, followed by a Knoevenagel reaction with diisopropyl malonate to form a diester .
Industrial Production Methods: While specific industrial production methods for this compound are not extensively documented, the synthesis methods used in laboratory settings can be adapted for larger-scale production. The use of palladium-catalyzed reactions and chiral ligands is crucial for maintaining the stereochemistry and yield of the compound .
Análisis De Reacciones Químicas
Types of Reactions: Bakkenolide A undergoes various chemical reactions, including:
Oxidation: This reaction can modify the lactone ring or other functional groups within the molecule.
Reduction: This reaction can reduce double bonds or other oxidized functional groups.
Substitution: This reaction can replace certain functional groups with others, potentially altering the biological activity of the compound.
Common Reagents and Conditions:
Oxidation: Common oxidizing agents include potassium permanganate and chromium trioxide.
Reduction: Common reducing agents include lithium aluminum hydride and sodium borohydride.
Substitution: Common reagents include halogens and nucleophiles under various conditions.
Major Products: The major products formed from these reactions depend on the specific reagents and conditions used. For example, oxidation may yield more oxidized derivatives of this compound, while reduction may yield more reduced forms of the compound.
Comparación Con Compuestos Similares
- Bakkenolide B
- Bakkenolide D
- Bakkenolide IIIa
- Bakkenolide IVa
Comparison: Bakkenolide A is unique among its counterparts due to its specific structural features and biological activities. While other bakkenolides also exhibit cytotoxic and antifeedant properties, this compound is particularly noted for its effectiveness against carcinoma cell lines and its potential as an insect antifeedant .
Propiedades
IUPAC Name |
(2R,3aR,7S,7aR)-7,7a-dimethyl-4'-methylidenespiro[3,3a,4,5,6,7-hexahydro-1H-indene-2,3'-oxolane]-2'-one | |
---|---|---|
Source | PubChem | |
URL | https://pubchem.ncbi.nlm.nih.gov | |
Description | Data deposited in or computed by PubChem | |
InChI |
InChI=1S/C15H22O2/c1-10-5-4-6-12-7-15(9-14(10,12)3)11(2)8-17-13(15)16/h10,12H,2,4-9H2,1,3H3/t10-,12+,14+,15+/m0/s1 | |
Source | PubChem | |
URL | https://pubchem.ncbi.nlm.nih.gov | |
Description | Data deposited in or computed by PubChem | |
InChI Key |
OVXAYHNZXBOVPV-QMGNLALYSA-N | |
Source | PubChem | |
URL | https://pubchem.ncbi.nlm.nih.gov | |
Description | Data deposited in or computed by PubChem | |
Canonical SMILES |
CC1CCCC2C1(CC3(C2)C(=C)COC3=O)C | |
Source | PubChem | |
URL | https://pubchem.ncbi.nlm.nih.gov | |
Description | Data deposited in or computed by PubChem | |
Isomeric SMILES |
C[C@H]1CCC[C@H]2[C@@]1(C[C@]3(C2)C(=C)COC3=O)C | |
Source | PubChem | |
URL | https://pubchem.ncbi.nlm.nih.gov | |
Description | Data deposited in or computed by PubChem | |
Molecular Formula |
C15H22O2 | |
Source | PubChem | |
URL | https://pubchem.ncbi.nlm.nih.gov | |
Description | Data deposited in or computed by PubChem | |
DSSTOX Substance ID |
DTXSID20173684 | |
Record name | Bakkenolide A | |
Source | EPA DSSTox | |
URL | https://comptox.epa.gov/dashboard/DTXSID20173684 | |
Description | DSSTox provides a high quality public chemistry resource for supporting improved predictive toxicology. | |
Molecular Weight |
234.33 g/mol | |
Source | PubChem | |
URL | https://pubchem.ncbi.nlm.nih.gov | |
Description | Data deposited in or computed by PubChem | |
CAS No. |
19906-72-0 | |
Record name | (+)-Bakkenolide A | |
Source | CAS Common Chemistry | |
URL | https://commonchemistry.cas.org/detail?cas_rn=19906-72-0 | |
Description | CAS Common Chemistry is an open community resource for accessing chemical information. Nearly 500,000 chemical substances from CAS REGISTRY cover areas of community interest, including common and frequently regulated chemicals, and those relevant to high school and undergraduate chemistry classes. This chemical information, curated by our expert scientists, is provided in alignment with our mission as a division of the American Chemical Society. | |
Explanation | The data from CAS Common Chemistry is provided under a CC-BY-NC 4.0 license, unless otherwise stated. | |
Record name | Bakkenolide A | |
Source | ChemIDplus | |
URL | https://pubchem.ncbi.nlm.nih.gov/substance/?source=chemidplus&sourceid=0019906720 | |
Description | ChemIDplus is a free, web search system that provides access to the structure and nomenclature authority files used for the identification of chemical substances cited in National Library of Medicine (NLM) databases, including the TOXNET system. | |
Record name | Bakkenolide A | |
Source | EPA DSSTox | |
URL | https://comptox.epa.gov/dashboard/DTXSID20173684 | |
Description | DSSTox provides a high quality public chemistry resource for supporting improved predictive toxicology. | |
Record name | BAKKENOLIDE A | |
Source | FDA Global Substance Registration System (GSRS) | |
URL | https://gsrs.ncats.nih.gov/ginas/app/beta/substances/T86ZPP5FMQ | |
Description | The FDA Global Substance Registration System (GSRS) enables the efficient and accurate exchange of information on what substances are in regulated products. Instead of relying on names, which vary across regulatory domains, countries, and regions, the GSRS knowledge base makes it possible for substances to be defined by standardized, scientific descriptions. | |
Explanation | Unless otherwise noted, the contents of the FDA website (www.fda.gov), both text and graphics, are not copyrighted. They are in the public domain and may be republished, reprinted and otherwise used freely by anyone without the need to obtain permission from FDA. Credit to the U.S. Food and Drug Administration as the source is appreciated but not required. | |
Retrosynthesis Analysis
AI-Powered Synthesis Planning: Our tool employs the Template_relevance Pistachio, Template_relevance Bkms_metabolic, Template_relevance Pistachio_ringbreaker, Template_relevance Reaxys, Template_relevance Reaxys_biocatalysis model, leveraging a vast database of chemical reactions to predict feasible synthetic routes.
One-Step Synthesis Focus: Specifically designed for one-step synthesis, it provides concise and direct routes for your target compounds, streamlining the synthesis process.
Accurate Predictions: Utilizing the extensive PISTACHIO, BKMS_METABOLIC, PISTACHIO_RINGBREAKER, REAXYS, REAXYS_BIOCATALYSIS database, our tool offers high-accuracy predictions, reflecting the latest in chemical research and data.
Strategy Settings
Precursor scoring | Relevance Heuristic |
---|---|
Min. plausibility | 0.01 |
Model | Template_relevance |
Template Set | Pistachio/Bkms_metabolic/Pistachio_ringbreaker/Reaxys/Reaxys_biocatalysis |
Top-N result to add to graph | 6 |
Feasible Synthetic Routes
ANone: The molecular formula of Bakkenolide A is C15H20O2, and its molecular weight is 232.32 g/mol.
ANone: Yes, researchers extensively use NMR spectroscopy (1H, 13C, COSY, HMQC, HMBC, NOESY) and mass spectrometry to characterize this compound and its derivatives [, , , ]. The chemical shifts of specific signals in 1H-NMR, particularly those for H-1 and H-9, are helpful in determining the location of substituents on the bakkenolide skeleton [].
ANone: Several total syntheses of this compound have been reported, highlighting different strategies:
- Intramolecular Diels-Alder Reaction: One approach utilizes a concise five-step synthesis involving sequential alkylations followed by a key intramolecular Diels-Alder reaction. This method also yields 7-epi-Bakkenolide A and novel 10-epi- and 7,10-diepithis compound stereoisomers as byproducts [, ].
- Ring Contraction Strategy: Another approach employs a diastereoselective route starting from the Wieland-Miescher ketone, featuring a ring contraction reaction, a stereoselective hydrogenation, and formation of a quaternary center [, ].
- Dichloroketene-Based Approach: A comprehensive method utilizes the cycloaddition of dichloroketene with dimethylcyclohexenes, leading to the synthesis of various bakkanes, including this compound and its derivatives [].
- Niobium Catalyzed Diels-Alder Reaction: A highly regioselective and stereoselective approach utilizes a niobium catalyzed Diels-Alder reaction to construct the core structure of bakkanes, subsequently leading to this compound [].
- Tsuji-Trost Pentadienylation: A regioselective Tsuji-Trost reaction is employed for the preparation of a key intermediate, 3-allyl-3-penta-2,4-dienyltetronic acid, ultimately leading to this compound [].
ANone: Yes, researchers have achieved the asymmetric synthesis of (+)-Bakkenolide A. Key steps include an asymmetric cyclization-carbonylation reaction to prepare a crucial intermediate and a diastereoselective construction of the spirolactone moiety []. Another approach utilizes chiral bis-oxazoline (box) ligands in an asymmetric intramolecular oxy-carbonylation reaction for the enantioselective synthesis of (+)-Bakkenolide A [].
ANone: Research indicates that this compound exhibits various biological activities:
- Insecticidal and Antifeedant Properties: this compound shows potent antifeedant and growth-inhibitory effects against several insect species, including stored product beetles and lepidopteran larvae [, , ].
- Anticancer Activity: While not as potent as Bakkenolide B, this compound exhibits some inhibitory effects on interleukin-2 production in human T cells, suggesting potential anti-cancer properties []. Another study showed this compound to have cytotoxic activity against several human cancer cell lines [].
- Neuroprotective Effects: Studies suggest that this compound, along with other bakkenolides, possesses neuroprotective effects against cerebral ischemic injury in rats. This activity is attributed to its antioxidant properties and ability to inhibit nuclear factor-κB (NF-κB) activation [, ].
ANone: While this compound itself isn't currently used in pharmaceuticals, its discovery and the exploration of its biological activities have contributed to the understanding of the bakkane family of compounds. Research on this compound and related bakkenolides, particularly their anti-inflammatory, neuroprotective, and anticancer properties, could lead to potential therapeutic applications in the future [, , ].
ANone: A study investigated the pharmacokinetics of this compound in rats after oral and intravenous administration. Results showed a relatively short elimination half-life and low oral bioavailability, suggesting further investigation into improving its pharmacokinetic profile is needed [].
ANone: Researchers commonly utilize high-performance liquid chromatography coupled with tandem mass spectrometry (LC-MS/MS) for the sensitive and accurate quantification of this compound in biological samples, such as rat plasma [, ].
ANone: Currently, there's limited information available regarding the specific environmental impact and degradation of this compound. Further research is needed to assess its potential ecotoxicological effects and develop strategies for mitigating any negative impacts.
ANone: Future research on this compound could focus on:
Descargo de responsabilidad e información sobre productos de investigación in vitro
Tenga en cuenta que todos los artículos e información de productos presentados en BenchChem están destinados únicamente con fines informativos. Los productos disponibles para la compra en BenchChem están diseñados específicamente para estudios in vitro, que se realizan fuera de organismos vivos. Los estudios in vitro, derivados del término latino "in vidrio", involucran experimentos realizados en entornos de laboratorio controlados utilizando células o tejidos. Es importante tener en cuenta que estos productos no se clasifican como medicamentos y no han recibido la aprobación de la FDA para la prevención, tratamiento o cura de ninguna condición médica, dolencia o enfermedad. Debemos enfatizar que cualquier forma de introducción corporal de estos productos en humanos o animales está estrictamente prohibida por ley. Es esencial adherirse a estas pautas para garantizar el cumplimiento de los estándares legales y éticos en la investigación y experimentación.