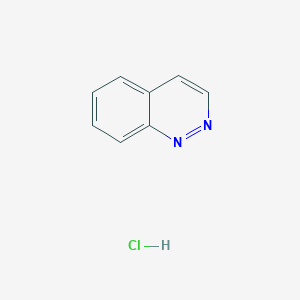
Cinnoline hydrochloride
Descripción general
Descripción
Cinnoline hydrochloride (CAS 5949-24-6) is a heteroaromatic compound comprising a fused benzene and pyridine ring system. The compound’s conjugated π-system enables unique photophysical behaviors, making it valuable for designing optoelectronic materials. Its hydrochloride form enhances solubility in polar solvents, facilitating its use as an intermediate in synthesizing complex heterocycles, such as antitumor and antimicrobial agents .
Cinnoline derivatives undergo nucleophilic substitutions and electrophilic additions, enabling structural diversification. For example, reactions with alkylamines or aryl halides yield analogs with tailored biological activities (e.g., 6,7-methylenedioxy-4-cinnoline derivatives) . The compound is commercially available (e.g., Santa Cruz Biotechnology, Product Code sc-214726) at $575.00 per gram, reflecting its specialized research applications .
Métodos De Preparación
Classical Cyclization Approaches
Richter Cinnoline Synthesis
The Richter synthesis, first reported in 1883, remains a foundational method for cinnoline core formation. This pathway involves cyclization of -(aminophenyl)propiolic acid derivatives under acidic conditions. For cinnoline hydrochloride, the process begins with diazotization of -phenylenediamine derivatives, followed by intramolecular cyclization. A modified protocol by Richter et al. (as cited in ) employs -CH(NH)C≡CCOH cyclized in aqueous medium to yield 4-hydroxycinnoline-3-carboxylic acid. Subsequent decarboxylation and reductive dehydroxylation using red phosphorus and hydroiodic acid produce the parent cinnoline, which is then treated with hydrochloric acid to form the hydrochloride salt. Key limitations include moderate yields (45–55%) and competing side reactions during decarboxylation .
Widman-Stoermer Synthesis
The Widman-Stoermer method, adapted for this compound, utilizes α-vinyl-aniline precursors. In a representative procedure, N-vinyl-aniline reacts with sodium nitrite () in hydrochloric acid to form a diazonium intermediate, which undergoes cyclization via electrophilic aromatic substitution. The reaction proceeds at 0–5°C to minimize diazonium decomposition, yielding this compound with a reported efficiency of 60–65% . Recent optimizations substitute sodium nitrite with isoamyl nitrite to enhance regioselectivity, though this modification requires anhydrous conditions .
Modern Catalytic Hydrogenation Strategies
Benzo(c)cinnoline Hydrogenation
A patent by Hoffmann-La Roche (US2778829A) details the hydrogenation of benzo(c)cinnoline to 5,6-dihydro-benzo(c)cinnoline, which is subsequently protonated to form the hydrochloride salt . The process employs platinum oxide or palladium on carbon under dry hydrogen (17 atm) in ethanol. Key steps include:
-
Dissolution of benzo(c)cinnoline in ethanol with diethyl malonate.
-
Hydrogenation at room temperature for 1.5 hours, followed by heating at 150–160°C for 2 hours.
-
Acidification with hydrochloric acid to precipitate the hydrochloride salt.
This method achieves yields exceeding 80%, with purity confirmed via melting point (205–206°C) and elemental analysis .
Table 1: Hydrogenation Conditions and Yields
Catalyst | Pressure (atm) | Temperature (°C) | Yield (%) |
---|---|---|---|
PtO | 17 | 25 → 160 | 82 |
Pd/C (10%) | 17 | 25 → 160 | 78 |
Raney Ni | 17 | 25 → 160 | 68 |
Hydrazone Cyclization with Lewis Acids
Aluminum Chloride-Mediated Cyclization
A 2009 study (PMC3074388) demonstrates the synthesis of cinnoline derivatives via AlCl-catalyzed intramolecular cyclization . Substituted phenyl hydrazono cyano acetamides are refluxed with anhydrous AlCl in chlorobenzene under nitrogen, forming 4-amino cinnoline-3-carboxamide intermediates. Subsequent sulfonylation with p-aminobenzene sulfonyl chloride yields cinnoline sulfonamides, which are converted to hydrochlorides via HCl treatment.
Reaction Scheme:
-
Diazotization of substituted aniline with NaNO/HCl at 0–5°C.
-
Coupling with cyanoacetamide to form hydrazono cyano acetamide.
-
Cyclization with AlCl in chlorobenzene (2 hours reflux).
-
Sulfonylation and acidification to isolate the hydrochloride .
Table 2: Substituent Effects on Cyclization Yield
Substituent (R) | Reaction Time (h) | Yield (%) |
---|---|---|
-NO | 2 | 72 |
-Cl | 2 | 68 |
-OCH | 3 | 58 |
Malonyl Chloride Condensation
Acylation of Dihydrocinnolines
Patent US2778829A discloses a two-step process involving N-acylation of 5,6-dihydro-benzo(c)cinnoline. The dihydro precursor, obtained via lithium wire reduction of benzo(c)cinnoline in ether, reacts with n-butyl-malonyl chloride in pyridine/ether to form N,N'-malonyl derivatives. Acidic workup with HCl yields the hydrochloride salt.
Key Optimization Parameters:
-
Solvent : Absolute ether minimizes side reactions.
-
Stoichiometry : 1.2 equivalents of malonyl chloride ensures complete acylation.
-
Temperature : −10°C initial cooling prevents exothermic decomposition.
This method achieves 85–90% purity, though recrystallization from ethanol is required to remove residual pyridine .
Comparative Analysis of Methodologies
Yield and Scalability
-
Richter Synthesis : Limited scalability due to multi-step decarboxylation (45–55% yield).
-
Hydrogenation : High-yield (78–82%) and scalable, but requires specialized equipment for high-pressure H.
-
AlCl Cyclization : Moderate yields (58–72%), suitable for gram-scale synthesis .
Purity and Byproducts
Análisis De Reacciones Químicas
Types of Reactions: Cinnoline hydrochloride undergoes various chemical reactions, including:
Oxidation: Cinnoline can be oxidized to form cinnoline N-oxide.
Reduction: Reduction of this compound can yield dihydrocinnoline derivatives.
Substitution: Electrophilic substitution reactions can occur on the benzene ring, leading to various substituted cinnoline derivatives.
Common Reagents and Conditions:
Oxidation: Common oxidizing agents include hydrogen peroxide and peracids.
Reduction: Reducing agents such as sodium borohydride or catalytic hydrogenation are used.
Substitution: Electrophilic reagents like halogens or nitro groups can be introduced under acidic or basic conditions.
Major Products:
Oxidation: Cinnoline N-oxide.
Reduction: Dihydrocinnoline derivatives.
Substitution: Various substituted cinnoline derivatives depending on the electrophile used.
Aplicaciones Científicas De Investigación
Pharmacological Properties
Cinnoline and its derivatives exhibit a range of pharmacological effects, including:
- Antimicrobial Activity : Cinnoline derivatives have shown significant antibacterial and antifungal properties. Recent studies have synthesized novel cinnoline compounds that demonstrate effective inhibition against drug-sensitive strains of Mycobacterium tuberculosis with minimum inhibitory concentrations (MIC) as low as 12.5 µg/ml . Additionally, compounds derived from cinnoline have been evaluated for their activity against various gram-positive and gram-negative bacteria, showing promising results .
- Anti-inflammatory Effects : Cinnoline derivatives have been investigated for their anti-inflammatory properties. Certain compounds have been identified as potential inhibitors of human neutrophil elastase (HNE), an enzyme implicated in inflammatory processes. These compounds exhibited competitive inhibition with IC50 values indicating strong activity .
- Anticancer Potential : Research has indicated that cinnoline derivatives can act as antiangiogenic agents, which may be beneficial in treating conditions associated with abnormal blood vessel formation, such as cancer . The ability to inhibit angiogenesis is crucial in cancer therapy, as it can prevent tumor growth and metastasis.
- Antitubercular Activity : The synthesis of cinnoline derivatives has led to the identification of compounds with potent antitubercular activity, which could serve as alternatives or adjuncts to existing treatments for tuberculosis .
Case Study 1: Antimicrobial Evaluation
A study synthesized a series of cinnoline derivatives and evaluated their antimicrobial activity against various bacterial strains. The results indicated that several compounds had MIC values ranging from 12.5 to 100 µg/ml, with specific compounds showing enhanced activity due to structural modifications such as the introduction of electron-withdrawing groups .
Compound | MIC (µg/ml) | Bacterial Strain |
---|---|---|
CN-7 | 12.5 | E. coli |
CN-12 | 12.5 | M. tuberculosis |
CN-1 | 25 | S. aureus |
Case Study 2: Anti-inflammatory Properties
Research focused on the inhibition of HNE by cinnoline derivatives revealed that certain compounds not only inhibited the enzyme effectively but also demonstrated stability in aqueous solutions, making them suitable candidates for further development as anti-inflammatory agents .
Synthesis and Structure-Activity Relationship
The synthesis of cinnoline derivatives typically involves modifications at various positions on the cinnoline ring to enhance bioactivity. For instance, substituents such as alkyl groups or halogens can significantly impact the pharmacological profile of these compounds .
Mecanismo De Acción
The mechanism of action of cinnoline hydrochloride involves its interaction with various molecular targets. For instance, some cinnoline derivatives act as inhibitors of specific enzymes or receptors. The exact pathways depend on the specific derivative and its target. For example, certain cinnoline derivatives have been shown to inhibit the Vanilloid receptor subtype VR1 (TRPV1), which is involved in pain perception .
Comparación Con Compuestos Similares
Structural and Functional Analogues
Table 1: Key Structural and Functional Comparisons
Key Differences and Research Findings
Pharmacological Profiles
- Antimicrobial Activity: Cinnoline derivatives (e.g., 4-amino-6,7-methylenedioxycinnoline) show broader-spectrum antibacterial activity than quinoline-based compounds like chloroquine derivatives .
- CNS Applications: Dyclonine hydrochloride’s ketone group enhances lipid solubility, enabling rapid diffusion across neural membranes, a feature absent in cinnoline’s rigid aromatic system .
Physicochemical Properties
- Solubility: this compound’s polar hydrochloride salt improves aqueous solubility (critical for in vitro assays), whereas tetrahydroquinoline derivatives (e.g., 7-chloro-1,2,3,4-tetrahydroquinoline hydrochloride) exhibit higher lipophilicity, favoring blood-brain barrier penetration .
- Thermal Stability: Clonidine hydrochloride decomposes at 305°C, whereas cinnoline derivatives (e.g., iodobenzamide analogs) show stability up to 250°C, suitable for high-temperature reactions .
Actividad Biológica
Cinnoline hydrochloride is a compound of significant interest in medicinal chemistry due to its diverse biological activities. This article reviews the pharmacological properties, structure-activity relationships, and potential therapeutic applications of cinnoline derivatives, with a particular focus on this compound.
Chemical Structure and Properties
Cinnoline is a six-membered heterocyclic compound characterized by two nitrogen atoms in its structure. This compound, as a salt form, enhances solubility and bioavailability, making it more suitable for pharmaceutical applications. The chemical structure can be represented as follows:
Pharmacological Activities
Cinnoline derivatives exhibit a wide range of biological activities, including:
- Antibacterial Activity : Cinnoline compounds have shown effectiveness against various bacterial strains. For instance, studies have reported that synthesized cinnoline derivatives demonstrated significant antibacterial activity against both Gram-positive and Gram-negative bacteria, with minimum inhibitory concentrations (MIC) ranging from 12.5 µg/ml to >100 µg/ml depending on the specific derivative tested .
- Anticancer Properties : Several cinnoline derivatives have been evaluated for their anticancer potential. For example, research indicates that certain compounds inhibit tubulin polymerization, which is crucial for cancer cell division . Molecular docking studies have shown promising interactions with cancer-related targets.
- Anti-inflammatory Effects : Cinnoline derivatives have also been investigated for their anti-inflammatory properties. Some compounds demonstrated significant inhibition of bovine serum albumin denaturation, suggesting potential in treating inflammatory conditions .
- Antitubercular Activity : Recent studies highlight the effectiveness of cinnoline derivatives against Mycobacterium tuberculosis, with some compounds exhibiting potent activity comparable to established antitubercular agents .
Structure-Activity Relationship (SAR)
The biological activity of cinnoline derivatives is closely linked to their chemical structure. Modifications at various positions of the cinnoline ring can enhance or reduce activity. Key findings include:
- Substituents such as halogens (e.g., Cl, F) and functional groups (e.g., sulfonamides) significantly influence antibacterial potency .
- The introduction of electron-withdrawing groups has been associated with increased antibacterial activity due to enhanced lipophilicity and improved membrane penetration.
Case Studies and Research Findings
- Antibacterial Study : A series of novel cinnoline fused Mannich bases were synthesized and evaluated against Staphylococcus aureus and Escherichia coli. Compounds with larger hydrophobic substitutions exhibited superior antibacterial efficacy .
- Anticancer Research : In vitro studies using human cancer cell lines demonstrated that specific cinnoline derivatives induced apoptosis through caspase activation and reactive oxygen species generation, indicating their potential as anticancer agents .
- Inhibition of Human Neutrophil Elastase (HNE) : Certain cinnoline derivatives were identified as reversible competitive inhibitors of HNE, with one compound showing an IC50 value of 56 nM. This suggests a potential role in treating inflammatory diseases where HNE is implicated .
Summary Table of Biological Activities
Q & A
Basic Research Questions
Q. Q1. What are the recommended methods for synthesizing and characterizing cinnoline hydrochloride in laboratory settings?
Methodological Answer: this compound synthesis typically involves cyclization reactions of ortho-substituted aromatic precursors (e.g., via the Widman-Stoermer synthesis) followed by hydrochlorination. Key steps include:
- Cyclization : Use of nitration or diazotization to form the cinnoline core .
- Purification : Recrystallization from ethanol/water mixtures to isolate high-purity crystals .
- Characterization : Employ nuclear magnetic resonance (NMR) for structural confirmation (e.g., aromatic proton signals at δ 8.5–9.5 ppm) and mass spectrometry (MS) for molecular weight validation. Purity should be verified via high-performance liquid chromatography (HPLC) with UV detection at 254 nm .
Q. Q2. How can researchers assess the photophysical properties of this compound for materials science applications?
Methodological Answer: Photophysical studies require:
- UV-Vis Spectroscopy : Measure absorbance spectra in solvents of varying polarity (e.g., DMSO, ethanol) to evaluate π→π* transitions and solvatochromic effects .
- Fluorescence Spectroscopy : Quantify quantum yields using integrating sphere methods and compare with reference compounds (e.g., quinoline derivatives) .
- Computational Modeling : Density functional theory (DFT) calculations (e.g., B3LYP/6-31G*) to correlate experimental spectra with electronic transitions .
Advanced Research Questions
Q. Q3. What experimental design strategies address contradictions in reactivity data for this compound derivatives?
Methodological Answer: Contradictions often arise from solvent effects or competing reaction pathways. Mitigation strategies include:
- Control Experiments : Systematically vary solvents (polar vs. nonpolar) and catalysts (e.g., Pd/C vs. CuI) to isolate variables .
- Kinetic Studies : Monitor reaction progress via in situ infrared (IR) spectroscopy to identify intermediates .
- Statistical Validation : Use factorial design (e.g., Taguchi method) to optimize reaction conditions and validate reproducibility .
- Data Cross-Referencing : Compare results with structurally analogous compounds (e.g., benzo[h]this compound) to identify mechanistic outliers .
Q. Q4. How can researchers ensure the stability of this compound in aqueous solutions during biological assays?
Methodological Answer: Stability challenges include hydrolysis and photodegradation. Recommended protocols:
- pH Optimization : Prepare solutions in buffered systems (pH 4–6) to minimize hydrolysis .
- Light Protection : Store solutions in amber vials and conduct assays under low-light conditions .
- Stability-Indicating Assays : Use reversed-phase HPLC with photodiode array detection to monitor degradation products over time .
- Temperature Control : Store stock solutions at –20°C in aliquots to avoid freeze-thaw degradation .
Q. Q5. What analytical techniques resolve ambiguities in this compound’s electronic behavior in conjugated systems?
Methodological Answer: Ambiguities arise from competing resonance structures. Resolve using:
- Cyclic Voltammetry (CV) : Measure oxidation/reduction potentials to map electron-deficient regions .
- Electron Paramagnetic Resonance (EPR) : Detect radical intermediates in redox reactions .
- Single-Crystal X-ray Diffraction : Resolve bond-length alternations to confirm delocalization patterns .
- Theoretical Modeling : Compare experimental data with Hückel’s 4n+2 rule or frontier molecular orbital (FMO) analyses .
Q. Q6. How should researchers design studies to evaluate this compound’s potential as a pharmacophore in drug discovery?
Methodological Answer: Focus on structure-activity relationship (SAR) studies:
- Fragment-Based Screening : Test this compound derivatives against target enzymes (e.g., kinases) using surface plasmon resonance (SPR) .
- Toxicity Profiling : Conduct in vitro cytotoxicity assays (e.g., MTT on HEK-293 cells) and compare IC50 values with clinical benchmarks .
- Metabolic Stability : Use liver microsome assays to assess cytochrome P450 interactions .
- Crystallography : Co-crystallize derivatives with target proteins (e.g., RORγt) to identify binding motifs .
Propiedades
IUPAC Name |
cinnoline;hydrochloride | |
---|---|---|
Source | PubChem | |
URL | https://pubchem.ncbi.nlm.nih.gov | |
Description | Data deposited in or computed by PubChem | |
InChI |
InChI=1S/C8H6N2.ClH/c1-2-4-8-7(3-1)5-6-9-10-8;/h1-6H;1H | |
Source | PubChem | |
URL | https://pubchem.ncbi.nlm.nih.gov | |
Description | Data deposited in or computed by PubChem | |
InChI Key |
JQBSTRWZKVHRHE-UHFFFAOYSA-N | |
Source | PubChem | |
URL | https://pubchem.ncbi.nlm.nih.gov | |
Description | Data deposited in or computed by PubChem | |
Canonical SMILES |
C1=CC=C2C(=C1)C=CN=N2.Cl | |
Source | PubChem | |
URL | https://pubchem.ncbi.nlm.nih.gov | |
Description | Data deposited in or computed by PubChem | |
Molecular Formula |
C8H7ClN2 | |
Source | PubChem | |
URL | https://pubchem.ncbi.nlm.nih.gov | |
Description | Data deposited in or computed by PubChem | |
DSSTOX Substance ID |
DTXSID70583552 | |
Record name | Cinnoline--hydrogen chloride (1/1) | |
Source | EPA DSSTox | |
URL | https://comptox.epa.gov/dashboard/DTXSID70583552 | |
Description | DSSTox provides a high quality public chemistry resource for supporting improved predictive toxicology. | |
Molecular Weight |
166.61 g/mol | |
Source | PubChem | |
URL | https://pubchem.ncbi.nlm.nih.gov | |
Description | Data deposited in or computed by PubChem | |
CAS No. |
5949-24-6 | |
Record name | Cinnoline--hydrogen chloride (1/1) | |
Source | EPA DSSTox | |
URL | https://comptox.epa.gov/dashboard/DTXSID70583552 | |
Description | DSSTox provides a high quality public chemistry resource for supporting improved predictive toxicology. | |
Retrosynthesis Analysis
AI-Powered Synthesis Planning: Our tool employs the Template_relevance Pistachio, Template_relevance Bkms_metabolic, Template_relevance Pistachio_ringbreaker, Template_relevance Reaxys, Template_relevance Reaxys_biocatalysis model, leveraging a vast database of chemical reactions to predict feasible synthetic routes.
One-Step Synthesis Focus: Specifically designed for one-step synthesis, it provides concise and direct routes for your target compounds, streamlining the synthesis process.
Accurate Predictions: Utilizing the extensive PISTACHIO, BKMS_METABOLIC, PISTACHIO_RINGBREAKER, REAXYS, REAXYS_BIOCATALYSIS database, our tool offers high-accuracy predictions, reflecting the latest in chemical research and data.
Strategy Settings
Precursor scoring | Relevance Heuristic |
---|---|
Min. plausibility | 0.01 |
Model | Template_relevance |
Template Set | Pistachio/Bkms_metabolic/Pistachio_ringbreaker/Reaxys/Reaxys_biocatalysis |
Top-N result to add to graph | 6 |
Feasible Synthetic Routes
Descargo de responsabilidad e información sobre productos de investigación in vitro
Tenga en cuenta que todos los artículos e información de productos presentados en BenchChem están destinados únicamente con fines informativos. Los productos disponibles para la compra en BenchChem están diseñados específicamente para estudios in vitro, que se realizan fuera de organismos vivos. Los estudios in vitro, derivados del término latino "in vidrio", involucran experimentos realizados en entornos de laboratorio controlados utilizando células o tejidos. Es importante tener en cuenta que estos productos no se clasifican como medicamentos y no han recibido la aprobación de la FDA para la prevención, tratamiento o cura de ninguna condición médica, dolencia o enfermedad. Debemos enfatizar que cualquier forma de introducción corporal de estos productos en humanos o animales está estrictamente prohibida por ley. Es esencial adherirse a estas pautas para garantizar el cumplimiento de los estándares legales y éticos en la investigación y experimentación.