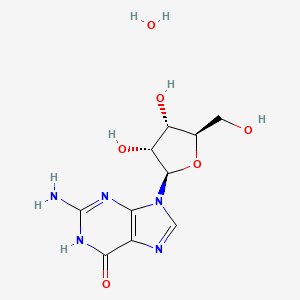
Guanosine Hydrate
Descripción general
Descripción
Guanosine Hydrate is a purine nucleoside that has different biological applications . It is a substrate for a wide range of G-protein coupled receptor (GPCR) GTPases, as well as cell signaling and cycling-associated guanine nucleotide exchange factors .
Synthesis Analysis
This compound can be synthesized and enhanced through its covalently grafting on polyvinyl alcohol (PVA), chitosan (CS), and cellulose (CL) .Molecular Structure Analysis
This compound has a molecular formula of C10H15N5O6 . It has an average mass of 301.256 Da and a monoisotopic mass of 301.102234 Da .Chemical Reactions Analysis
This compound can form complexes with water molecules, which can profoundly influence its physicochemical properties . The addition of water molecules increases the experimental adiabatic detachment (ADE) and vertical detachment energy (VDE) by 0.5−0.1 eV, depending on the cluster size .Physical And Chemical Properties Analysis
This compound is a white, crystalline powder with no odor and a mild saline taste . It is very soluble in acetic acid, slightly soluble in water, and insoluble in ethanol, diethyl ether, benzene, and chloroform .Aplicaciones Científicas De Investigación
Neuroprotective and Antioxidant Properties
Neuroprotection in Astroglial Cells
Guanosine, a guanine-based purine, has shown antioxidant properties and protective effects against oxidative damage in C6 astroglial cells. It effectively mitigates cytotoxicity and modulates glial, oxidative, and inflammatory responses through the activation of the heme oxygenase 1 pathway, offering significant neuroprotection (Quincozes-Santos et al., 2014).
Antidepressant-Like Effects and Hippocampal Protection
Guanosine has demonstrated antidepressant-like properties in animal models, associated with the modulation of hippocampal oxidative stress. It effectively reduces behavioral alterations and oxidative damage in the hippocampus, indicative of its potential therapeutic applications in mood disorders (Bettio et al., 2014).
Supramolecular Applications and Material Science
Gelation and Hydrogel Formation
Guanosine derivatives can form stable supramolecular hydrogels through guanine quartet (G-quartet) formation in the presence of metal cations. These hydrogels demonstrate significant potential for controlled release of bioactive substances and have applications in material science and drug delivery (Sreenivasachary & Lehn, 2005).
Controlled Release of Bioactive Molecules
Guanosine-based hydrogels are capable of entrapping and selectively releasing various biologically active molecules, like acyclovir, vitamin C, and vancomycin. This implies potential applications in targeted drug delivery and tissue engineering (Sreenivasachary & Lehn, 2008).
Phase Transformation and Crystallization
- Phase Transformation in Continuous Crystallization: The phase transformation of guanosine 5-monophosphate (GMP), closely related to guanosine hydrate, has been studied in continuous crystallizers. Such studies provide insights into optimizing crystallization processes in industrial and pharmaceutical applications (Nguyen et al., 2011).
Neuropharmacology and Brain Disorders
Potential in Treating Neurodegenerative Diseases
Research suggests that guanosine plays a role in modulating neurotrophic and neuroprotective effects, showing promise in treating neurodegenerative diseases like Parkinson's and Alzheimer's, and in response to brain injuries (Lanznaster et al., 2016).
Antiparkinsonian Efficacy
Guanosine has shown effectiveness in reversing motor impairments in rodent models of movement disorder, suggesting its potential as an antiparkinsonian agent. This finding is significant for developing new treatments for Parkinson’s disease (Massari et al., 2017).
Mecanismo De Acción
Guanosine is required for an RNA splicing reaction in mRNA, where a “self-splicing” intron removes itself from the mRNA message by cutting at both ends, re-ligating, and leaving just the exons on either side to be translated into protein . It can also be phosphorylated to become GMP (guanosine monophosphate), cGMP (cyclic guanosine monophosphate), GDP (guanosine diphosphate) and GTP (guanosine triphosphate) which are factors in signal transduction pathways .
Safety and Hazards
Direcciones Futuras
There is a growing interest in the self-assembly of Guanosine and its derivatives for devising programmable supramolecular biomaterials including hydrogels . Future research may focus on preparing Guanosine-based supramolecular hydrogels with longer lifespan, unique physicochemical properties, and biological activities for a broad range of biological and medical applications .
Propiedades
IUPAC Name |
2-amino-9-[(2R,3R,4S,5R)-3,4-dihydroxy-5-(hydroxymethyl)oxolan-2-yl]-1H-purin-6-one;hydrate | |
---|---|---|
Source | PubChem | |
URL | https://pubchem.ncbi.nlm.nih.gov | |
Description | Data deposited in or computed by PubChem | |
InChI |
InChI=1S/C10H13N5O5.H2O/c11-10-13-7-4(8(19)14-10)12-2-15(7)9-6(18)5(17)3(1-16)20-9;/h2-3,5-6,9,16-18H,1H2,(H3,11,13,14,19);1H2/t3-,5-,6-,9-;/m1./s1 | |
Source | PubChem | |
URL | https://pubchem.ncbi.nlm.nih.gov | |
Description | Data deposited in or computed by PubChem | |
InChI Key |
YCHNAJLCEKPFHB-GWTDSMLYSA-N | |
Source | PubChem | |
URL | https://pubchem.ncbi.nlm.nih.gov | |
Description | Data deposited in or computed by PubChem | |
Canonical SMILES |
C1=NC2=C(N1C3C(C(C(O3)CO)O)O)N=C(NC2=O)N.O | |
Source | PubChem | |
URL | https://pubchem.ncbi.nlm.nih.gov | |
Description | Data deposited in or computed by PubChem | |
Isomeric SMILES |
C1=NC2=C(N1[C@H]3[C@@H]([C@@H]([C@H](O3)CO)O)O)N=C(NC2=O)N.O | |
Source | PubChem | |
URL | https://pubchem.ncbi.nlm.nih.gov | |
Description | Data deposited in or computed by PubChem | |
Molecular Formula |
C10H15N5O6 | |
Source | PubChem | |
URL | https://pubchem.ncbi.nlm.nih.gov | |
Description | Data deposited in or computed by PubChem | |
DSSTOX Substance ID |
DTXSID50901952 | |
Record name | Guanosine hydrate (1:1) | |
Source | EPA DSSTox | |
URL | https://comptox.epa.gov/dashboard/DTXSID50901952 | |
Description | DSSTox provides a high quality public chemistry resource for supporting improved predictive toxicology. | |
Molecular Weight |
301.26 g/mol | |
Source | PubChem | |
URL | https://pubchem.ncbi.nlm.nih.gov | |
Description | Data deposited in or computed by PubChem | |
CAS RN |
1143525-19-2 | |
Record name | Guanosine hydrate (1:1) | |
Source | EPA DSSTox | |
URL | https://comptox.epa.gov/dashboard/DTXSID50901952 | |
Description | DSSTox provides a high quality public chemistry resource for supporting improved predictive toxicology. | |
Retrosynthesis Analysis
AI-Powered Synthesis Planning: Our tool employs the Template_relevance Pistachio, Template_relevance Bkms_metabolic, Template_relevance Pistachio_ringbreaker, Template_relevance Reaxys, Template_relevance Reaxys_biocatalysis model, leveraging a vast database of chemical reactions to predict feasible synthetic routes.
One-Step Synthesis Focus: Specifically designed for one-step synthesis, it provides concise and direct routes for your target compounds, streamlining the synthesis process.
Accurate Predictions: Utilizing the extensive PISTACHIO, BKMS_METABOLIC, PISTACHIO_RINGBREAKER, REAXYS, REAXYS_BIOCATALYSIS database, our tool offers high-accuracy predictions, reflecting the latest in chemical research and data.
Strategy Settings
Precursor scoring | Relevance Heuristic |
---|---|
Min. plausibility | 0.01 |
Model | Template_relevance |
Template Set | Pistachio/Bkms_metabolic/Pistachio_ringbreaker/Reaxys/Reaxys_biocatalysis |
Top-N result to add to graph | 6 |
Feasible Synthetic Routes
Descargo de responsabilidad e información sobre productos de investigación in vitro
Tenga en cuenta que todos los artículos e información de productos presentados en BenchChem están destinados únicamente con fines informativos. Los productos disponibles para la compra en BenchChem están diseñados específicamente para estudios in vitro, que se realizan fuera de organismos vivos. Los estudios in vitro, derivados del término latino "in vidrio", involucran experimentos realizados en entornos de laboratorio controlados utilizando células o tejidos. Es importante tener en cuenta que estos productos no se clasifican como medicamentos y no han recibido la aprobación de la FDA para la prevención, tratamiento o cura de ninguna condición médica, dolencia o enfermedad. Debemos enfatizar que cualquier forma de introducción corporal de estos productos en humanos o animales está estrictamente prohibida por ley. Es esencial adherirse a estas pautas para garantizar el cumplimiento de los estándares legales y éticos en la investigación y experimentación.