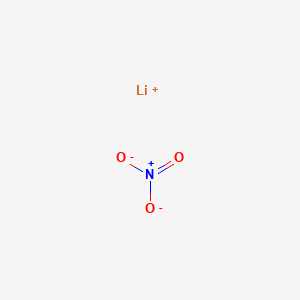
Nitrato de litio
Descripción general
Descripción
Lithium nitrate is a nitrate of lithium. It is an oxidizing agent used in the manufacture of red-colored fireworks and flares. Nitrite is a toxic compound known to cause methemoglobinemia. (L1137, L1630)
Aplicaciones Científicas De Investigación
Almacenamiento de energía en baterías de litio
LiNO₃ es un aditivo bien conocido en las baterías de litio-azufre, donde juega un papel crucial en la regulación de la interfase de electrolito sólido (SEI). Esto ayuda a suprimir eficazmente el transporte redox de los polisulfuros, lo que lleva a una mayor estabilidad de la SEI y un mejor rendimiento del ciclo . Los iones nitrato de LiNO₃ contribuyen a la formación de una capa SEI estable, que es esencial para la longevidad y la eficiencia de la batería.
Agente estabilizador en baterías de litio-azufre
Se ha demostrado que la adición de LiNO₃ en las baterías de litio-azufre reduce la evolución de gases, un problema común que afecta la estabilidad y la seguridad de la batería . Al actuar como un aditivo estabilizador, LiNO₃ ayuda a minimizar los efectos perjudiciales del transporte de polisulfuros y la descomposición del electrolito, mejorando así el rendimiento general y la seguridad de estas baterías.
Almacenamiento de energía solar
El nitrato de litio se ha propuesto como un medio para almacenar energía solar. Se puede utilizar una lente de Fresnel para fundir LiNO₃ sólido, que luego funciona como una "batería solar", permitiendo que el calor almacenado se redistribuya posteriormente por convección . Esta aplicación es particularmente prometedora para áreas con abundante luz solar y la necesidad de soluciones energéticas sostenibles.
Materiales aeroespaciales
En la industria aeroespacial, LiNO₃ encuentra aplicaciones debido a sus propiedades que contribuyen al desarrollo de materiales que pueden resistir condiciones extremas . Su estabilidad térmica y química lo hace adecuado para su uso en entornos que requieren que los materiales funcionen de manera confiable en una amplia gama de temperaturas y presiones.
Mecanismo De Acción
Target of Action
Lithium nitrate is an inorganic compound with the formula LiNO3 . It is the lithium salt of nitric acid In the context of lithium-sulfur batteries, lithium nitrate acts as a stabilizing additive .
Mode of Action
Lithium nitrate’s mode of action is largely dependent on its application. In lithium-sulfur batteries, it is used as an electrolyte additive. The prevailing understanding is that lithium nitrate reacts with the metallic lithium anode . This reaction helps to stabilize the battery and improve its performance .
Biochemical Pathways
It is known that lithium has many widely varying biochemical and phenomenological effects, suggesting that a systems biology approach is required to understand its action . Multiple lines of evidence point to lithium as a significant factor in the development of neurodegenerative disease .
Pharmacokinetics
The pharmacokinetics of lithium, in general, is linear within the dose regimen used in clinical practice . Lithium has an extremely narrow therapeutic window . .
Result of Action
The result of lithium nitrate’s action is largely dependent on its application. In lithium-sulfur batteries, lithium nitrate helps to reduce gassing, a common issue in these batteries . This results in improved stability and performance of the battery .
Action Environment
The action environment of lithium nitrate can influence its efficacy and stability. For instance, in the context of thermal storage, the hydrated form of lithium nitrate, lithium nitrate trihydrate, can be used for thermal energy storage at its melt temperature of 303.3 K . This property has led to proposals of using lithium nitrate as a medium to store heat collected from the sun for cooking .
Safety and Hazards
Direcciones Futuras
Lithium Nitrate has been proposed as a medium to store heat collected from the sun for cooking . A Fresnel lens would be used to melt solid lithium nitrate, which would then function as a “solar battery”, allowing heat to be redistributed later by convection . Lithium-ion batteries are also a significant area of research, with lithium nitrate playing a crucial role .
Análisis Bioquímico
Biochemical Properties
Lithium Nitrate has been found to interact with various biomolecules in biological systems. For instance, it has been observed to affect the activities of enzymes such as aspartate transaminase (AST), alanine transaminase (ALT), and lactate dehydrogenase (LDH) . The nature of these interactions often leads to changes in the physiological status of the organism .
Cellular Effects
Lithium Nitrate has significant effects on various types of cells and cellular processes. It influences cell function by affecting cellular metabolism and gene expression . For example, it has been found to cause alterations in gross hematological values, leading to conditions such as hypochromic anemia and leucocytosis .
Molecular Mechanism
At the molecular level, Lithium Nitrate exerts its effects through binding interactions with biomolecules and changes in gene expression . It has been observed to stimulate transamination reactions in hepatic and renal tissues .
Temporal Effects in Laboratory Settings
In laboratory settings, the effects of Lithium Nitrate have been observed to change over time. For instance, it has been found to cause significant alterations in the general physiological status of rats when administered orally over a period of 7 weeks .
Dosage Effects in Animal Models
The effects of Lithium Nitrate vary with different dosages in animal models. For example, small doses of Lithium Nitrate have been found to induce toxicity in rats . At high doses, it can cause adverse effects such as ocular side effects, polyuria, polydipsia, and loss of body mass .
Metabolic Pathways
Lithium Nitrate is involved in various metabolic pathways. It interacts with enzymes and cofactors, leading to changes in metabolic flux or metabolite levels . For instance, it has been found to cause a significant increase in serum calcium and phosphorus levels .
Transport and Distribution
Given its observed effects on cellular function and metabolism, it is likely that it interacts with various transporters or binding proteins .
Subcellular Localization
Given its observed effects on cellular function and metabolism, it is likely that it is directed to specific compartments or organelles within the cell .
Propiedades
{ "Design of the Synthesis Pathway": "The synthesis pathway for Lithium nitrate involves the reaction between Lithium hydroxide and Nitric acid.", "Starting Materials": [ "Lithium hydroxide (LiOH)", "Nitric acid (HNO3)" ], "Reaction": [ "Dissolve Lithium hydroxide in water to form Lithium hydroxide solution", "Add Nitric acid slowly to the Lithium hydroxide solution with stirring", "Heat the mixture to evaporate the water and concentrate the Lithium nitrate solution", "Cool the solution to room temperature and collect the Lithium nitrate crystals by filtration", "Dry the crystals to obtain pure Lithium nitrate" ] } | |
Número CAS |
7790-69-4 |
Fórmula molecular |
LiNO3 |
Peso molecular |
69.0 g/mol |
Nombre IUPAC |
lithium;nitrate |
InChI |
InChI=1S/Li.NO3/c;2-1(3)4/q+1;-1 |
Clave InChI |
IIPYXGDZVMZOAP-UHFFFAOYSA-N |
SMILES |
[Li+].[N+](=O)([O-])[O-] |
SMILES canónico |
[Li+].[N+](=O)([O-])[O-] |
7790-69-4 | |
Descripción física |
Lithium nitrate appears as a white to light yellow colored crystalline solid. Denser than water. Contact with the material may cause irritation to skin, eyes, and mucous membranes. May be toxic by ingestion. May cause the acceleration of the burning of combustible materials. Prolonged exposure to heat or flames may result in an explosion. DryPowder, Liquid; Liquid; OtherSolid |
Pictogramas |
Oxidizer; Irritant |
Sinónimos |
LiNO3 |
Origen del producto |
United States |
Synthesis routes and methods
Procedure details
Descargo de responsabilidad e información sobre productos de investigación in vitro
Tenga en cuenta que todos los artículos e información de productos presentados en BenchChem están destinados únicamente con fines informativos. Los productos disponibles para la compra en BenchChem están diseñados específicamente para estudios in vitro, que se realizan fuera de organismos vivos. Los estudios in vitro, derivados del término latino "in vidrio", involucran experimentos realizados en entornos de laboratorio controlados utilizando células o tejidos. Es importante tener en cuenta que estos productos no se clasifican como medicamentos y no han recibido la aprobación de la FDA para la prevención, tratamiento o cura de ninguna condición médica, dolencia o enfermedad. Debemos enfatizar que cualquier forma de introducción corporal de estos productos en humanos o animales está estrictamente prohibida por ley. Es esencial adherirse a estas pautas para garantizar el cumplimiento de los estándares legales y éticos en la investigación y experimentación.