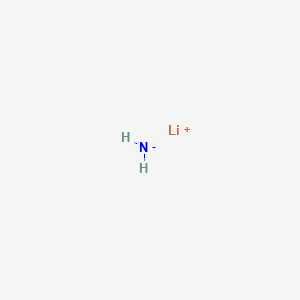
Lithium amide
Descripción general
Descripción
Mecanismo De Acción
Target of Action
Lithium amide, or lithium azanide, is an inorganic compound with the chemical formula LiNH2 . It is a strong base and is very reactive . It is known to target enzymes that have magnesium as a co-factor .
Mode of Action
This compound can be made by treating lithium metal with liquid ammonia . The reaction is as follows:
2Li+2NH3→2LiNH2+H22 Li + 2 NH_3 \rightarrow 2 LiNH_2 + H_2 2Li+2NH3→2LiNH2+H2
This reaction results in the formation of this compound and hydrogen gas .
Biochemical Pathways
This compound is involved in various biochemical pathways. For instance, it plays a role in the Claisen condensation and Aldol condensation, as well as the alkylation of nitriles, ketones, amines, and alkynes . It is also used in the synthesis of ethynyl compounds and antihistamines .
Result of Action
The result of the action of this compound is largely dependent on the specific reaction it is involved in. For instance, in the synthesis of mixed metal amides, the solid-state reaction of this compound and sodium amide leads to the synthesis of the mixed metal amides .
Action Environment
This compound reacts vigorously with water to generate gaseous NH3 .
Análisis Bioquímico
Biochemical Properties
Lithium amide plays a significant role in biochemical reactions due to its strong basicity and weak nucleophilicity . It interacts with various enzymes, proteins, and other biomolecules, primarily through deprotonation reactions. For instance, this compound can deprotonate amines to form lithium amides, which are crucial intermediates in organic synthesis . The interaction between this compound and biomolecules often involves the formation of covalent bonds, which can alter the structure and function of the target molecules .
Cellular Effects
This compound influences various cellular processes by affecting cell signaling pathways, gene expression, and cellular metabolism . It can modulate the activity of enzymes involved in metabolic pathways, leading to changes in the levels of metabolites and overall cellular function . Additionally, this compound has been shown to impact cell signaling pathways by altering the phosphorylation status of key signaling proteins, thereby influencing gene expression and cellular responses .
Molecular Mechanism
The molecular mechanism of this compound involves its ability to form covalent bonds with biomolecules, leading to enzyme inhibition or activation and changes in gene expression . This compound can bind to the active sites of enzymes, inhibiting their activity by forming stable complexes . This binding can also induce conformational changes in the enzymes, affecting their catalytic efficiency . Furthermore, this compound can influence gene expression by modulating the activity of transcription factors and other regulatory proteins .
Temporal Effects in Laboratory Settings
In laboratory settings, the effects of this compound can change over time due to its stability and degradation properties . This compound is relatively stable at room temperature but decomposes into ammonia and lithium imide upon heating . Long-term exposure to this compound can lead to alterations in cellular function, including changes in metabolic activity and gene expression . These temporal effects are crucial for understanding the compound’s behavior in both in vitro and in vivo studies.
Dosage Effects in Animal Models
The effects of this compound vary with different dosages in animal models . At low doses, this compound can modulate enzyme activity and cellular metabolism without causing significant toxicity . At high doses, this compound can induce toxic effects, including cellular damage and disruption of metabolic pathways . Threshold effects have been observed, where a specific dosage range leads to optimal biochemical activity, while higher doses result in adverse effects .
Metabolic Pathways
This compound is involved in various metabolic pathways, primarily through its interactions with enzymes and cofactors . It can deprotonate amines to form lithium amides, which are intermediates in organic synthesis and metabolic reactions . This compound can also affect metabolic flux by altering the activity of key enzymes, leading to changes in the levels of metabolites and overall metabolic balance .
Transport and Distribution
Within cells and tissues, this compound is transported and distributed through interactions with transporters and binding proteins . These interactions facilitate the localization and accumulation of this compound in specific cellular compartments . The transport and distribution of this compound are crucial for its biochemical activity and effects on cellular function .
Subcellular Localization
This compound exhibits specific subcellular localization, which influences its activity and function . It can be directed to particular compartments or organelles through targeting signals and post-translational modifications . The subcellular localization of this compound is essential for its role in biochemical reactions and its impact on cellular processes .
Métodos De Preparación
Synthetic Routes and Reaction Conditions: Lithium amide can be synthesized by treating lithium metal with liquid ammonia. The reaction proceeds as follows: [ 2 \text{Li} + 2 \text{NH}_3 \rightarrow 2 \text{LiNH}_2 + \text{H}_2 ] This reaction typically requires low temperatures to control the reactivity and prevent the decomposition of this compound .
Industrial Production Methods: In industrial settings, this compound is often produced by reacting lithium metal with gaseous ammonia at elevated temperatures. This method is advantageous as it avoids the release of hydrogen, which is a common byproduct in other preparation methods .
Análisis De Reacciones Químicas
Types of Reactions: Lithium amide undergoes various types of chemical reactions, including:
Decomposition: Upon heating, this compound decomposes into ammonia and lithium imide.
Reduction: this compound can be used to reduce esters and other carbonyl-containing compounds.
Common Reagents and Conditions:
Reduction: this compound can be used in combination with lithium aluminum hydride for the reduction of amides to amines.
Major Products Formed:
Ammonia and Lithium Imide: Formed during the decomposition of this compound.
Aplicaciones Científicas De Investigación
Lithium amide has a wide range of applications in scientific research, including:
Organic Synthesis: Used as a strong base and nucleophile in various organic reactions.
Hydrogen Storage: this compound, when mixed with lithium hydride, is used in hydrogen storage systems.
Dye Synthesis: Used in the production of dyes that display large Stokes shifts.
Comparación Con Compuestos Similares
- Sodium Amide (NaNH₂)
- Potassium Amide (KNH₂)
- Lithium Diisopropylamide (LDA)
- Lithium Tetramethylpiperidide (LiTMP)
- Lithium Hexamethyldisilazide (LiHMDS)
Comparison: Lithium amide is unique due to its high basicity and relatively low nucleophilicity compared to other lithium amides like lithium diisopropylamide and lithium hexamethyldisilazide . This makes this compound particularly useful in reactions where strong basicity is required without significant nucleophilic interference. Additionally, this compound’s ability to decompose into ammonia and lithium imide upon heating is a distinctive feature that is not commonly observed in other similar compounds .
Propiedades
IUPAC Name |
lithium;azanide | |
---|---|---|
Source | PubChem | |
URL | https://pubchem.ncbi.nlm.nih.gov | |
Description | Data deposited in or computed by PubChem | |
InChI |
InChI=1S/Li.H2N/h;1H2/q+1;-1 | |
Source | PubChem | |
URL | https://pubchem.ncbi.nlm.nih.gov | |
Description | Data deposited in or computed by PubChem | |
InChI Key |
AFRJJFRNGGLMDW-UHFFFAOYSA-N | |
Source | PubChem | |
URL | https://pubchem.ncbi.nlm.nih.gov | |
Description | Data deposited in or computed by PubChem | |
Canonical SMILES |
[Li+].[NH2-] | |
Source | PubChem | |
URL | https://pubchem.ncbi.nlm.nih.gov | |
Description | Data deposited in or computed by PubChem | |
Molecular Formula |
H2LiN | |
Record name | LITHIUM AMIDE | |
Source | CAMEO Chemicals | |
URL | https://cameochemicals.noaa.gov/chemical/991 | |
Description | CAMEO Chemicals is a chemical database designed for people who are involved in hazardous material incident response and planning. CAMEO Chemicals contains a library with thousands of datasheets containing response-related information and recommendations for hazardous materials that are commonly transported, used, or stored in the United States. CAMEO Chemicals was developed by the National Oceanic and Atmospheric Administration's Office of Response and Restoration in partnership with the Environmental Protection Agency's Office of Emergency Management. | |
Explanation | CAMEO Chemicals and all other CAMEO products are available at no charge to those organizations and individuals (recipients) responsible for the safe handling of chemicals. However, some of the chemical data itself is subject to the copyright restrictions of the companies or organizations that provided the data. | |
Source | PubChem | |
URL | https://pubchem.ncbi.nlm.nih.gov | |
Description | Data deposited in or computed by PubChem | |
DSSTOX Substance ID |
DTXSID7064815 | |
Record name | Lithium amide | |
Source | EPA DSSTox | |
URL | https://comptox.epa.gov/dashboard/DTXSID7064815 | |
Description | DSSTox provides a high quality public chemistry resource for supporting improved predictive toxicology. | |
Molecular Weight |
23.0 g/mol | |
Source | PubChem | |
URL | https://pubchem.ncbi.nlm.nih.gov | |
Description | Data deposited in or computed by PubChem | |
Physical Description |
Lithium amide is a white crystalline powder with an odor of ammonia. Denser than water., White to colorless crystals with an ammonia-like odor; [HSDB] | |
Record name | LITHIUM AMIDE | |
Source | CAMEO Chemicals | |
URL | https://cameochemicals.noaa.gov/chemical/991 | |
Description | CAMEO Chemicals is a chemical database designed for people who are involved in hazardous material incident response and planning. CAMEO Chemicals contains a library with thousands of datasheets containing response-related information and recommendations for hazardous materials that are commonly transported, used, or stored in the United States. CAMEO Chemicals was developed by the National Oceanic and Atmospheric Administration's Office of Response and Restoration in partnership with the Environmental Protection Agency's Office of Emergency Management. | |
Explanation | CAMEO Chemicals and all other CAMEO products are available at no charge to those organizations and individuals (recipients) responsible for the safe handling of chemicals. However, some of the chemical data itself is subject to the copyright restrictions of the companies or organizations that provided the data. | |
Record name | Lithium amide | |
Source | Haz-Map, Information on Hazardous Chemicals and Occupational Diseases | |
URL | https://haz-map.com/Agents/1618 | |
Description | Haz-Map® is an occupational health database designed for health and safety professionals and for consumers seeking information about the adverse effects of workplace exposures to chemical and biological agents. | |
Explanation | Copyright (c) 2022 Haz-Map(R). All rights reserved. Unless otherwise indicated, all materials from Haz-Map are copyrighted by Haz-Map(R). No part of these materials, either text or image may be used for any purpose other than for personal use. Therefore, reproduction, modification, storage in a retrieval system or retransmission, in any form or by any means, electronic, mechanical or otherwise, for reasons other than personal use, is strictly prohibited without prior written permission. | |
Solubility |
Slightly soluble in ethanol, SLIGHTLY SOL IN LIQ AMMONIA, ALCOHOL, Insoluble in anhydrous ether, benzene, toluene | |
Record name | LITHIUM AMIDE | |
Source | Hazardous Substances Data Bank (HSDB) | |
URL | https://pubchem.ncbi.nlm.nih.gov/source/hsdb/649 | |
Description | The Hazardous Substances Data Bank (HSDB) is a toxicology database that focuses on the toxicology of potentially hazardous chemicals. It provides information on human exposure, industrial hygiene, emergency handling procedures, environmental fate, regulatory requirements, nanomaterials, and related areas. The information in HSDB has been assessed by a Scientific Review Panel. | |
Density |
1.18 g/cu cm | |
Record name | LITHIUM AMIDE | |
Source | Hazardous Substances Data Bank (HSDB) | |
URL | https://pubchem.ncbi.nlm.nih.gov/source/hsdb/649 | |
Description | The Hazardous Substances Data Bank (HSDB) is a toxicology database that focuses on the toxicology of potentially hazardous chemicals. It provides information on human exposure, industrial hygiene, emergency handling procedures, environmental fate, regulatory requirements, nanomaterials, and related areas. The information in HSDB has been assessed by a Scientific Review Panel. | |
Color/Form |
Tetragonal crystals, White crystalline powder | |
CAS No. |
7782-89-0 | |
Record name | LITHIUM AMIDE | |
Source | CAMEO Chemicals | |
URL | https://cameochemicals.noaa.gov/chemical/991 | |
Description | CAMEO Chemicals is a chemical database designed for people who are involved in hazardous material incident response and planning. CAMEO Chemicals contains a library with thousands of datasheets containing response-related information and recommendations for hazardous materials that are commonly transported, used, or stored in the United States. CAMEO Chemicals was developed by the National Oceanic and Atmospheric Administration's Office of Response and Restoration in partnership with the Environmental Protection Agency's Office of Emergency Management. | |
Explanation | CAMEO Chemicals and all other CAMEO products are available at no charge to those organizations and individuals (recipients) responsible for the safe handling of chemicals. However, some of the chemical data itself is subject to the copyright restrictions of the companies or organizations that provided the data. | |
Record name | Lithium amide | |
Source | CAS Common Chemistry | |
URL | https://commonchemistry.cas.org/detail?cas_rn=7782-89-0 | |
Description | CAS Common Chemistry is an open community resource for accessing chemical information. Nearly 500,000 chemical substances from CAS REGISTRY cover areas of community interest, including common and frequently regulated chemicals, and those relevant to high school and undergraduate chemistry classes. This chemical information, curated by our expert scientists, is provided in alignment with our mission as a division of the American Chemical Society. | |
Explanation | The data from CAS Common Chemistry is provided under a CC-BY-NC 4.0 license, unless otherwise stated. | |
Record name | Lithium amide | |
Source | ChemIDplus | |
URL | https://pubchem.ncbi.nlm.nih.gov/substance/?source=chemidplus&sourceid=0007782890 | |
Description | ChemIDplus is a free, web search system that provides access to the structure and nomenclature authority files used for the identification of chemical substances cited in National Library of Medicine (NLM) databases, including the TOXNET system. | |
Record name | Lithium amide (Li(NH2)) | |
Source | EPA Chemicals under the TSCA | |
URL | https://www.epa.gov/chemicals-under-tsca | |
Description | EPA Chemicals under the Toxic Substances Control Act (TSCA) collection contains information on chemicals and their regulations under TSCA, including non-confidential content from the TSCA Chemical Substance Inventory and Chemical Data Reporting. | |
Record name | Lithium amide | |
Source | EPA DSSTox | |
URL | https://comptox.epa.gov/dashboard/DTXSID7064815 | |
Description | DSSTox provides a high quality public chemistry resource for supporting improved predictive toxicology. | |
Record name | Lithium amide | |
Source | European Chemicals Agency (ECHA) | |
URL | https://echa.europa.eu/substance-information/-/substanceinfo/100.029.062 | |
Description | The European Chemicals Agency (ECHA) is an agency of the European Union which is the driving force among regulatory authorities in implementing the EU's groundbreaking chemicals legislation for the benefit of human health and the environment as well as for innovation and competitiveness. | |
Explanation | Use of the information, documents and data from the ECHA website is subject to the terms and conditions of this Legal Notice, and subject to other binding limitations provided for under applicable law, the information, documents and data made available on the ECHA website may be reproduced, distributed and/or used, totally or in part, for non-commercial purposes provided that ECHA is acknowledged as the source: "Source: European Chemicals Agency, http://echa.europa.eu/". Such acknowledgement must be included in each copy of the material. ECHA permits and encourages organisations and individuals to create links to the ECHA website under the following cumulative conditions: Links can only be made to webpages that provide a link to the Legal Notice page. | |
Record name | LITHIUM AMIDE | |
Source | FDA Global Substance Registration System (GSRS) | |
URL | https://gsrs.ncats.nih.gov/ginas/app/beta/substances/7393OMU9LK | |
Description | The FDA Global Substance Registration System (GSRS) enables the efficient and accurate exchange of information on what substances are in regulated products. Instead of relying on names, which vary across regulatory domains, countries, and regions, the GSRS knowledge base makes it possible for substances to be defined by standardized, scientific descriptions. | |
Explanation | Unless otherwise noted, the contents of the FDA website (www.fda.gov), both text and graphics, are not copyrighted. They are in the public domain and may be republished, reprinted and otherwise used freely by anyone without the need to obtain permission from FDA. Credit to the U.S. Food and Drug Administration as the source is appreciated but not required. | |
Record name | LITHIUM AMIDE | |
Source | Hazardous Substances Data Bank (HSDB) | |
URL | https://pubchem.ncbi.nlm.nih.gov/source/hsdb/649 | |
Description | The Hazardous Substances Data Bank (HSDB) is a toxicology database that focuses on the toxicology of potentially hazardous chemicals. It provides information on human exposure, industrial hygiene, emergency handling procedures, environmental fate, regulatory requirements, nanomaterials, and related areas. The information in HSDB has been assessed by a Scientific Review Panel. | |
Melting Point |
Starts to decompose at 320 °C and melts at 375 °C | |
Record name | LITHIUM AMIDE | |
Source | Hazardous Substances Data Bank (HSDB) | |
URL | https://pubchem.ncbi.nlm.nih.gov/source/hsdb/649 | |
Description | The Hazardous Substances Data Bank (HSDB) is a toxicology database that focuses on the toxicology of potentially hazardous chemicals. It provides information on human exposure, industrial hygiene, emergency handling procedures, environmental fate, regulatory requirements, nanomaterials, and related areas. The information in HSDB has been assessed by a Scientific Review Panel. | |
Q1: How do lithium amides interact with ketones?
A: Lithium amides, like LDA, are widely recognized for their ability to deprotonate ketones at the α-carbon, leading to the formation of enolates. [, , , ] This deprotonation is often highly regioselective, favoring the less sterically hindered site. []
Q2: Can lithium amides react with substrates other than ketones?
A: Yes, lithium amides demonstrate reactivity with various functional groups. They can deprotonate imines, [] rearrange epoxides to allylic alcohols, [, , ] and metalate organometallic complexes, particularly tricarbonyl(η6-arene)chromium(0) complexes. [, ] Additionally, they can react with certain phosphorus- or sulfur-containing compounds. []
Q3: How do chiral lithium amides induce asymmetry in reactions?
A: Chiral lithium amides, derived from chiral diamines, can differentiate between enantiotopic protons during deprotonation. [, ] This selectivity arises from the chiral environment created by the lithium amide, favoring the abstraction of one specific proton over its enantiotopic counterpart, leading to enantiomerically enriched products. [, , , , , , ]
Q4: What are the downstream effects of this compound deprotonation?
A: The enolates and other carbanions generated by this compound deprotonation are valuable intermediates in organic synthesis. [, ] They can undergo further reactions, such as alkylation, aldol condensation, and Michael addition, to construct complex molecules with defined stereochemistry. [, , , , ]
Q5: What is the molecular formula and weight of this compound?
A: The molecular formula of this compound is LiNH2, and its molecular weight is 22.96 g/mol. []
Q6: How does the structure of lithium amides affect their reactivity?
A: The aggregation state of lithium amides significantly influences their reactivity. [, , ] They typically exist as dimers or higher aggregates in solution, impacting their steric environment and Lewis acidity. [, , , , ] Solvent polarity and the presence of additives can alter the aggregation state and, consequently, their reactivity. [, , , , ]
Q7: What spectroscopic techniques are used to characterize this compound structures?
A: Nuclear magnetic resonance (NMR) spectroscopy, particularly 6Li and 15N NMR, is extensively used to elucidate the solution structures of lithium amides. [, , , , , ] Additionally, techniques like Infrared (IR) spectroscopy and X-ray diffraction contribute to understanding their structural characteristics. [, , ]
Q8: Are there any compatibility issues with lithium amides in organic synthesis?
A: Lithium amides are strong bases and can react with protic solvents. Therefore, they are typically used in aprotic solvents like tetrahydrofuran (THF) or diethyl ether. [, , , ] Additionally, their compatibility with certain functional groups in the substrate requires careful consideration. []
Q9: Can chiral lithium amides be used in catalytic amounts for asymmetric deprotonation?
A: Yes, certain reactions, like epoxide rearrangements, can be performed with sub-stoichiometric amounts of chiral lithium amides in the presence of an achiral bulk base, like LDA. [, ] The chiral this compound acts as a catalyst, regenerating in the process. [, ]
Q10: How does the solvent affect the enantioselectivity of chiral this compound-mediated reactions?
A: The solvent plays a crucial role in determining the enantioselectivity of these reactions. [, ] Different solvents can lead to changes in the aggregation state of the this compound, influencing the chiral environment during deprotonation and ultimately impacting the enantiomeric excess of the product. [, ]
Q11: How is computational chemistry employed in studying lithium amides?
A: Density Functional Theory (DFT) calculations are frequently used to study the electronic structure, stability, and bonding of this compound, lithium imide, and lithium hydride in the context of hydrogen storage. [, ] These calculations help in understanding the thermodynamics and reaction pathways involved. [, ]
Q12: Are there any QSAR models developed for lithium amides?
A: While QSAR models specifically for lithium amides are not extensively reported in the provided research, molecular mechanics calculations, such as MM3, have been employed to study this compound compounds. [] These calculations focus on understanding molecular structures, conformational energies, and vibrational spectra. []
Q13: How do modifications to the chiral amine structure affect the enantioselectivity of this compound bases?
A: Structural modifications of the chiral amine used to generate the this compound base significantly impact the enantioselectivity of the reaction. [, , , , ] Factors like the steric bulk around the nitrogen atom, the presence of additional coordinating groups, and the rigidity of the chiral scaffold can all influence the chiral environment and impact the enantiomeric excess of the product. [, , , , ]
Q14: How can the stability of lithium amides be improved?
A: The stability of lithium amides can be enhanced by storing them under an inert atmosphere, such as nitrogen or argon, to prevent reaction with moisture and oxygen. [] Additionally, using low temperatures during storage and handling can minimize decomposition. []
Q15: Are there any alternatives to lithium amides for similar applications?
A: Yes, alternative strong bases like potassium bis(trimethylsilyl)amide (KHMDS) and sodium bis(trimethylsilyl)amide (NaHMDS) can be employed for similar deprotonation reactions. [] The choice of base often depends on factors such as substrate, reaction conditions, and desired selectivity. []
Q16: How are lithium amides typically handled and disposed of in a laboratory setting?
A: Lithium amides are moisture-sensitive and should be handled under an inert atmosphere using appropriate techniques. Disposal typically involves quenching with a suitable reagent, such as isopropanol, followed by careful neutralization and disposal according to local regulations. []
Q17: What are some essential resources for conducting research on lithium amides?
A: Essential resources include access to well-equipped synthetic chemistry laboratories with facilities for handling air- and moisture-sensitive reagents. Additionally, access to spectroscopic techniques such as NMR, IR, and X-ray diffraction is crucial for characterizing this compound compounds. []
Q18: When did chiral lithium amides emerge as reagents for asymmetric synthesis?
A: Chiral lithium amides began to be utilized in asymmetric deprotonation reactions around 1980. [] This marked a significant advancement in the field of asymmetric synthesis, providing access to enantioenriched building blocks for various natural products and pharmaceuticals. [, ]
Q19: How has the study of lithium amides contributed to other fields?
A: Research on lithium amides extends beyond traditional organic synthesis. Their use in hydrogen storage systems, particularly the this compound/lithium hydride system, highlights their potential in materials science and energy applications. [, , , ] This interdisciplinary research has fostered collaborations between chemists, material scientists, and engineers. []
Descargo de responsabilidad e información sobre productos de investigación in vitro
Tenga en cuenta que todos los artículos e información de productos presentados en BenchChem están destinados únicamente con fines informativos. Los productos disponibles para la compra en BenchChem están diseñados específicamente para estudios in vitro, que se realizan fuera de organismos vivos. Los estudios in vitro, derivados del término latino "in vidrio", involucran experimentos realizados en entornos de laboratorio controlados utilizando células o tejidos. Es importante tener en cuenta que estos productos no se clasifican como medicamentos y no han recibido la aprobación de la FDA para la prevención, tratamiento o cura de ninguna condición médica, dolencia o enfermedad. Debemos enfatizar que cualquier forma de introducción corporal de estos productos en humanos o animales está estrictamente prohibida por ley. Es esencial adherirse a estas pautas para garantizar el cumplimiento de los estándares legales y éticos en la investigación y experimentación.