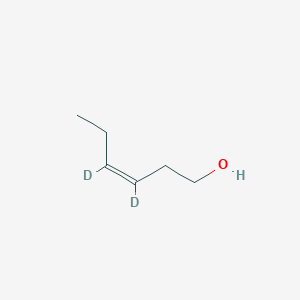
(Z)-3,4-Dideuteriohex-3-en-1-ol
- Haga clic en CONSULTA RÁPIDA para recibir una cotización de nuestro equipo de expertos.
- Con productos de calidad a un precio COMPETITIVO, puede centrarse más en su investigación.
Descripción general
Descripción
(Z)-3,4-Dideuteriohex-3-en-1-ol, also known as 3,4-dideuterio-3-hexen-1-ol, is a deuterated analog of the natural compound (Z)-3-hexen-1-ol. It is a colorless liquid with a grassy odor and is commonly used in scientific research for its unique properties.
Mecanismo De Acción
The mechanism of action of (Z)-3,4-Dideuteriohex-3-en-1-ol is similar to that of (Z)-3-hexen-1-ol. It is believed to interact with olfactory receptors in insects and trigger a behavioral response. In plants, (Z)-3,4-Dideuteriohex-3-en-1-ol may act as a signaling molecule to induce defense responses.
Biochemical and Physiological Effects:
Studies have shown that (Z)-3,4-Dideuteriohex-3-en-1-ol has similar biochemical and physiological effects to (Z)-3-hexen-1-ol. It has been shown to induce the expression of genes involved in plant defense and to attract insect predators that feed on herbivores.
Ventajas Y Limitaciones Para Experimentos De Laboratorio
The use of (Z)-3,4-Dideuteriohex-3-en-1-ol in scientific research provides several advantages. Its deuterated nature allows for easy identification and quantification in experiments, and its purity ensures accurate results. However, its high cost and limited availability may pose limitations for some experiments.
Direcciones Futuras
Future research on (Z)-3,4-Dideuteriohex-3-en-1-ol could focus on its potential applications in agriculture and pest control. It could also be used to investigate the role of (Z)-3-hexen-1-ol in plant-microbe interactions and to develop new methods for detecting and quantifying this compound in various environments.
In conclusion, (Z)-3,4-Dideuteriohex-3-en-1-ol is a valuable tool in scientific research for investigating the metabolic pathways and physiological effects of (Z)-3-hexen-1-ol. Its unique properties provide advantages for experiments, and future research could lead to new applications and discoveries.
Métodos De Síntesis
The synthesis of (Z)-3,4-Dideuteriohex-3-en-1-ol involves the reaction of deuterated acetylene with a deuterated allyl alcohol in the presence of a catalyst. This method yields a high purity product with a high degree of deuterium incorporation.
Aplicaciones Científicas De Investigación
(Z)-3,4-Dideuteriohex-3-en-1-ol is commonly used in scientific research as a tool to investigate the metabolic pathways and physiological effects of (Z)-3-hexen-1-ol. It has been used in studies to examine the biosynthesis of (Z)-3-hexen-1-ol in plants and the mechanisms of its perception in insects.
Propiedades
IUPAC Name |
(Z)-3,4-dideuteriohex-3-en-1-ol |
Source
|
---|---|---|
Source | PubChem | |
URL | https://pubchem.ncbi.nlm.nih.gov | |
Description | Data deposited in or computed by PubChem | |
InChI |
InChI=1S/C6H12O/c1-2-3-4-5-6-7/h3-4,7H,2,5-6H2,1H3/b4-3-/i3D,4D |
Source
|
Source | PubChem | |
URL | https://pubchem.ncbi.nlm.nih.gov | |
Description | Data deposited in or computed by PubChem | |
InChI Key |
UFLHIIWVXFIJGU-KKLCAENNSA-N |
Source
|
Source | PubChem | |
URL | https://pubchem.ncbi.nlm.nih.gov | |
Description | Data deposited in or computed by PubChem | |
Canonical SMILES |
CCC=CCCO |
Source
|
Source | PubChem | |
URL | https://pubchem.ncbi.nlm.nih.gov | |
Description | Data deposited in or computed by PubChem | |
Isomeric SMILES |
[2H]/C(=C(\[2H])/CCO)/CC |
Source
|
Source | PubChem | |
URL | https://pubchem.ncbi.nlm.nih.gov | |
Description | Data deposited in or computed by PubChem | |
Molecular Formula |
C6H12O |
Source
|
Source | PubChem | |
URL | https://pubchem.ncbi.nlm.nih.gov | |
Description | Data deposited in or computed by PubChem | |
Molecular Weight |
102.17 g/mol |
Source
|
Source | PubChem | |
URL | https://pubchem.ncbi.nlm.nih.gov | |
Description | Data deposited in or computed by PubChem | |
Product Name |
(Z)-3,4-Dideuteriohex-3-en-1-ol |
Descargo de responsabilidad e información sobre productos de investigación in vitro
Tenga en cuenta que todos los artículos e información de productos presentados en BenchChem están destinados únicamente con fines informativos. Los productos disponibles para la compra en BenchChem están diseñados específicamente para estudios in vitro, que se realizan fuera de organismos vivos. Los estudios in vitro, derivados del término latino "in vidrio", involucran experimentos realizados en entornos de laboratorio controlados utilizando células o tejidos. Es importante tener en cuenta que estos productos no se clasifican como medicamentos y no han recibido la aprobación de la FDA para la prevención, tratamiento o cura de ninguna condición médica, dolencia o enfermedad. Debemos enfatizar que cualquier forma de introducción corporal de estos productos en humanos o animales está estrictamente prohibida por ley. Es esencial adherirse a estas pautas para garantizar el cumplimiento de los estándares legales y éticos en la investigación y experimentación.