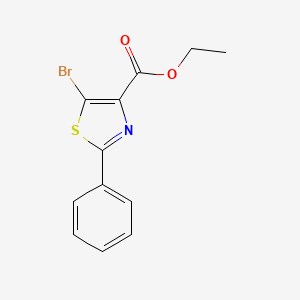
Ethyl 5-bromo-2-phenylthiazole-4-carboxylate
Descripción general
Descripción
Ethyl 5-bromo-2-phenylthiazole-4-carboxylate is a useful research compound. Its molecular formula is C12H10BrNO2S and its molecular weight is 312.18 g/mol. The purity is usually 95%.
BenchChem offers high-quality this compound suitable for many research applications. Different packaging options are available to accommodate customers' requirements. Please inquire for more information about this compound including the price, delivery time, and more detailed information at info@benchchem.com.
Aplicaciones Científicas De Investigación
Synthesis of Heterocycles
Ethyl 5-bromo-2-phenylthiazole-4-carboxylate serves as a precursor in the synthesis of various heterocyclic compounds, demonstrating the versatility of this compound in organic synthesis. For example, a study illustrated its utility in synthesizing [1,2,3]Triazolo[1,5-a]quinoline derivatives through a series of reactions that highlight its reactivity and potential for generating complex structures (Pokhodylo & Obushak, 2019).
Methodological Advancements
Advancements in synthetic methodologies using this compound have been reported, which streamline the synthesis of thiazole derivatives. A notable example includes an efficient one-pot synthesis technique that offers a practical and green approach to generating 2-substituted-4-methylthiazole-5-carboxylates from readily available materials, demonstrating improved yields under mild conditions (Meng, Wang, Zheng, Dou, & Guo, 2014).
Photophysical and Photochemical Properties
The photophysical and photochemical properties of thiazole derivatives synthesized from this compound have been explored, revealing their potential in photodynamic therapy and as photosensitizers. Research into tandem photoarylation-photoisomerization of halothiazoles to synthesize ethyl 2-arylthiazole-5-carboxylates has shed light on their photophysical behaviors and singlet oxygen activation capabilities, indicating their utility in medical and material sciences (Amati, Belviso, D’Auria, Lelj, Racioppi, & Viggiani, 2010).
Antimicrobial Applications
While focusing on the synthesis and structural analysis, some derivatives of this compound have been studied for their antimicrobial properties. Synthetic modifications and subsequent testing against various bacterial and fungal strains have identified compounds with significant antimicrobial activity, underscoring the potential of these derivatives in developing new antibacterial and antifungal agents (Desai, Bhatt, & Joshi, 2019).
Mecanismo De Acción
Pharmacokinetics
Ethyl 5-bromo-2-phenylthiazole-4-carboxylate exhibits high gastrointestinal absorption and is permeable to the blood-brain barrier . The compound is predicted to inhibit several cytochrome P450 enzymes, including CYP1A2, CYP2C19, and CYP2C9 . These enzymes are involved in drug metabolism, and their inhibition can affect the compound’s pharmacokinetic properties. The compound’s logP values suggest it is moderately lipophilic , which can influence its distribution within the body.
Action Environment
The action, efficacy, and stability of this compound can be influenced by various environmental factors. For instance, its storage temperature should be between 2-8°C in a dry environment . Furthermore, its solubility can affect its distribution in the body and its interactions with targets . More research is needed to fully understand how environmental factors influence the action of this compound.
Análisis Bioquímico
Biochemical Properties
Ethyl 5-bromo-2-phenylthiazole-4-carboxylate plays a significant role in various biochemical reactions due to its ability to interact with specific enzymes, proteins, and other biomolecules. This compound is known to inhibit certain enzymes, such as cytochrome P450 enzymes, including CYP1A2, CYP2C19, and CYP2C9 . These interactions are primarily due to the compound’s structural features, which allow it to bind to the active sites of these enzymes, thereby inhibiting their catalytic activity. Additionally, this compound may interact with other proteins and biomolecules through hydrogen bonding, hydrophobic interactions, and van der Waals forces, further influencing various biochemical pathways.
Cellular Effects
This compound has been shown to affect various types of cells and cellular processes. This compound can influence cell function by modulating cell signaling pathways, gene expression, and cellular metabolism. For instance, this compound may alter the expression of genes involved in metabolic pathways, leading to changes in cellular energy production and utilization . Additionally, this compound can impact cell signaling pathways by inhibiting or activating specific kinases and phosphatases, thereby affecting cellular responses to external stimuli.
Molecular Mechanism
The molecular mechanism of action of this compound involves its binding interactions with various biomolecules. This compound can inhibit enzyme activity by binding to the active sites of enzymes, such as cytochrome P450 enzymes, and preventing substrate access . Additionally, this compound may modulate gene expression by interacting with transcription factors and other regulatory proteins, leading to changes in the transcriptional activity of specific genes. These molecular interactions contribute to the compound’s overall biochemical and cellular effects.
Temporal Effects in Laboratory Settings
In laboratory settings, the effects of this compound can change over time due to its stability, degradation, and long-term impact on cellular function. This compound is relatively stable when stored under appropriate conditions, such as in a sealed container at 2-8°C . Over extended periods, this compound may undergo degradation, leading to a decrease in its potency and effectiveness. Long-term exposure to this compound in in vitro or in vivo studies may result in cumulative effects on cellular function, including alterations in gene expression and metabolic activity.
Dosage Effects in Animal Models
The effects of this compound can vary with different dosages in animal models. At lower doses, this compound may exhibit beneficial effects, such as enzyme inhibition and modulation of cellular processes. At higher doses, this compound may cause toxic or adverse effects, including cytotoxicity and disruption of normal cellular function . Threshold effects may also be observed, where a specific dosage range is required to achieve the desired biochemical and cellular outcomes.
Metabolic Pathways
This compound is involved in various metabolic pathways, primarily through its interactions with enzymes and cofactors. This compound can influence metabolic flux by inhibiting key enzymes in metabolic pathways, such as cytochrome P450 enzymes . Additionally, this compound may affect metabolite levels by altering the activity of enzymes involved in the synthesis and degradation of specific metabolites. These interactions contribute to the compound’s overall impact on cellular metabolism.
Transport and Distribution
The transport and distribution of this compound within cells and tissues are influenced by its interactions with transporters and binding proteins. This compound may be transported across cell membranes by specific transporters, such as organic anion transporters, and distributed within various cellular compartments . Additionally, this compound may bind to intracellular proteins, affecting its localization and accumulation within cells.
Subcellular Localization
This compound exhibits specific subcellular localization, which can influence its activity and function. This compound may be directed to particular cellular compartments or organelles through targeting signals or post-translational modifications . For example, this compound may localize to the endoplasmic reticulum or mitochondria, where it can interact with enzymes and other biomolecules involved in metabolic processes. The subcellular localization of this compound is crucial for its overall biochemical and cellular effects.
Propiedades
IUPAC Name |
ethyl 5-bromo-2-phenyl-1,3-thiazole-4-carboxylate | |
---|---|---|
Source | PubChem | |
URL | https://pubchem.ncbi.nlm.nih.gov | |
Description | Data deposited in or computed by PubChem | |
InChI |
InChI=1S/C12H10BrNO2S/c1-2-16-12(15)9-10(13)17-11(14-9)8-6-4-3-5-7-8/h3-7H,2H2,1H3 | |
Source | PubChem | |
URL | https://pubchem.ncbi.nlm.nih.gov | |
Description | Data deposited in or computed by PubChem | |
InChI Key |
ONAJVAODOMRERW-UHFFFAOYSA-N | |
Source | PubChem | |
URL | https://pubchem.ncbi.nlm.nih.gov | |
Description | Data deposited in or computed by PubChem | |
Canonical SMILES |
CCOC(=O)C1=C(SC(=N1)C2=CC=CC=C2)Br | |
Source | PubChem | |
URL | https://pubchem.ncbi.nlm.nih.gov | |
Description | Data deposited in or computed by PubChem | |
Molecular Formula |
C12H10BrNO2S | |
Source | PubChem | |
URL | https://pubchem.ncbi.nlm.nih.gov | |
Description | Data deposited in or computed by PubChem | |
DSSTOX Substance ID |
DTXSID30659424 | |
Record name | Ethyl 5-bromo-2-phenyl-1,3-thiazole-4-carboxylate | |
Source | EPA DSSTox | |
URL | https://comptox.epa.gov/dashboard/DTXSID30659424 | |
Description | DSSTox provides a high quality public chemistry resource for supporting improved predictive toxicology. | |
Molecular Weight |
312.18 g/mol | |
Source | PubChem | |
URL | https://pubchem.ncbi.nlm.nih.gov | |
Description | Data deposited in or computed by PubChem | |
CAS No. |
914347-21-0 | |
Record name | Ethyl 5-bromo-2-phenyl-1,3-thiazole-4-carboxylate | |
Source | EPA DSSTox | |
URL | https://comptox.epa.gov/dashboard/DTXSID30659424 | |
Description | DSSTox provides a high quality public chemistry resource for supporting improved predictive toxicology. | |
Retrosynthesis Analysis
AI-Powered Synthesis Planning: Our tool employs the Template_relevance Pistachio, Template_relevance Bkms_metabolic, Template_relevance Pistachio_ringbreaker, Template_relevance Reaxys, Template_relevance Reaxys_biocatalysis model, leveraging a vast database of chemical reactions to predict feasible synthetic routes.
One-Step Synthesis Focus: Specifically designed for one-step synthesis, it provides concise and direct routes for your target compounds, streamlining the synthesis process.
Accurate Predictions: Utilizing the extensive PISTACHIO, BKMS_METABOLIC, PISTACHIO_RINGBREAKER, REAXYS, REAXYS_BIOCATALYSIS database, our tool offers high-accuracy predictions, reflecting the latest in chemical research and data.
Strategy Settings
Precursor scoring | Relevance Heuristic |
---|---|
Min. plausibility | 0.01 |
Model | Template_relevance |
Template Set | Pistachio/Bkms_metabolic/Pistachio_ringbreaker/Reaxys/Reaxys_biocatalysis |
Top-N result to add to graph | 6 |
Feasible Synthetic Routes
Descargo de responsabilidad e información sobre productos de investigación in vitro
Tenga en cuenta que todos los artículos e información de productos presentados en BenchChem están destinados únicamente con fines informativos. Los productos disponibles para la compra en BenchChem están diseñados específicamente para estudios in vitro, que se realizan fuera de organismos vivos. Los estudios in vitro, derivados del término latino "in vidrio", involucran experimentos realizados en entornos de laboratorio controlados utilizando células o tejidos. Es importante tener en cuenta que estos productos no se clasifican como medicamentos y no han recibido la aprobación de la FDA para la prevención, tratamiento o cura de ninguna condición médica, dolencia o enfermedad. Debemos enfatizar que cualquier forma de introducción corporal de estos productos en humanos o animales está estrictamente prohibida por ley. Es esencial adherirse a estas pautas para garantizar el cumplimiento de los estándares legales y éticos en la investigación y experimentación.