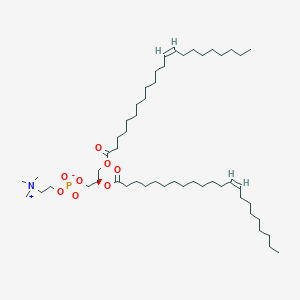
1,2-Dierucoyl-sn-glycero-3-fosfocolina
Descripción general
Descripción
1,2-Dierucoyl-sn-glycero-3-phosphocholine is a phospholipid containing the unsaturated long-chain fatty acid, erucic acid, at the sn-1 and sn-2 positions. This compound is commonly used in the generation of micelles, liposomes, and other types of artificial membranes . It is a key component in the study of lipid bilayers and membrane dynamics due to its unique structural properties.
Aplicaciones Científicas De Investigación
1,2-Dierucoyl-sn-glycero-3-phosphocholine is extensively used in scientific research due to its role in the formation of lipid bilayers and liposomes. Some key applications include:
Chemistry: Used to study the properties of lipid bilayers and membrane dynamics.
Biology: Employed in the preparation of liposomes for drug delivery and as model membranes to study membrane protein interactions.
Medicine: Utilized in the development of liposomal drug delivery systems and in the study of lipid-related diseases.
Industry: Applied in the formulation of cosmetics and personal care products due to its emollient properties
Mecanismo De Acción
Target of Action
1,2-Dierucoyl-sn-glycero-3-phosphocholine (DEPC) is a phospholipid that primarily targets the lipid bilayer of cell membranes . It is a key component of liposomes, which are used in various biomedical applications .
Mode of Action
DEPC interacts with its target by integrating into the lipid bilayer of cell membranes. This interaction can alter the properties of the membrane, such as its fluidity and permeability . In liposomes, DEPC can encapsulate and protect various bioactive compounds, enhancing their delivery to specific cells or tissues .
Biochemical Pathways
For example, the glucose oxidase (GO) in the DEPC liposome shows high activity .
Pharmacokinetics
The pharmacokinetics of DEPC are largely determined by its incorporation into liposomes. Liposomes can enhance the bioavailability of encapsulated compounds by protecting them from degradation and facilitating their transport across biological barriers
Result of Action
The primary result of DEPC’s action is the formation of stable liposomes that can deliver encapsulated compounds to specific cells or tissues . By altering the properties of cell membranes, DEPC may also influence cellular processes such as signal transduction and endocytosis .
Action Environment
The action of DEPC can be influenced by various environmental factors. For example, the stability and efficacy of DEPC liposomes can be affected by factors such as temperature, pH, and the presence of certain ions
Análisis Bioquímico
Biochemical Properties
1,2-Dierucoyl-sn-glycero-3-phosphocholine plays a crucial role in biochemical reactions, particularly in the formation and stability of lipid bilayers. It interacts with enzymes such as glucose oxidase, which shows high activity when encapsulated in liposomes containing 1,2-Dierucoyl-sn-glycero-3-phosphocholine . This interaction is essential for studying the properties of lipid bilayers and the behavior of enzymes within these structures.
Cellular Effects
1,2-Dierucoyl-sn-glycero-3-phosphocholine influences various cellular processes, including cell signaling pathways, gene expression, and cellular metabolism. It is used to prepare liposomes that can affect cell function by altering the lipid composition of cell membranes . This alteration can impact cell signaling pathways and gene expression, leading to changes in cellular metabolism and overall cell function.
Molecular Mechanism
At the molecular level, 1,2-Dierucoyl-sn-glycero-3-phosphocholine exerts its effects through binding interactions with biomolecules and enzyme activation. The compound’s long-chain fatty acids allow it to integrate into lipid bilayers, affecting membrane fluidity and enzyme activity . This integration can lead to changes in gene expression and cellular responses, making it a valuable tool for studying molecular mechanisms in biochemical research.
Temporal Effects in Laboratory Settings
In laboratory settings, the effects of 1,2-Dierucoyl-sn-glycero-3-phosphocholine can change over time. The compound is stable when stored at -20°C for up to three years in powder form and up to six months in solvent at -80°C . Over time, its stability and degradation can influence its long-term effects on cellular function, which are observed in both in vitro and in vivo studies.
Dosage Effects in Animal Models
The effects of 1,2-Dierucoyl-sn-glycero-3-phosphocholine vary with different dosages in animal models. At lower doses, it can enhance the activity of enzymes like glucose oxidase, while higher doses may lead to toxic or adverse effects . Understanding the dosage effects is crucial for optimizing its use in biochemical research and ensuring its safety in experimental settings.
Metabolic Pathways
1,2-Dierucoyl-sn-glycero-3-phosphocholine is involved in metabolic pathways related to lipid metabolism. It interacts with enzymes and cofactors that regulate lipid synthesis and degradation . These interactions can affect metabolic flux and metabolite levels, providing insights into the role of phospholipids in cellular metabolism.
Transport and Distribution
Within cells and tissues, 1,2-Dierucoyl-sn-glycero-3-phosphocholine is transported and distributed through interactions with transporters and binding proteins . These interactions influence its localization and accumulation in specific cellular compartments, affecting its overall activity and function.
Subcellular Localization
1,2-Dierucoyl-sn-glycero-3-phosphocholine is localized in specific subcellular compartments, such as lipid bilayers and membrane-bound organelles . Its activity and function are influenced by targeting signals and post-translational modifications that direct it to these compartments, making it a valuable tool for studying subcellular processes.
Métodos De Preparación
Synthetic Routes and Reaction Conditions: 1,2-Dierucoyl-sn-glycero-3-phosphocholine is synthesized through a series of chemical reactions involving erucic acid and phosphocholine. The synthesis typically involves the esterification of erucic acid with glycerol, followed by the phosphorylation of the resulting diacylglycerol with phosphocholine .
Industrial Production Methods: Industrial production of 1,2-Dierucoyl-sn-glycero-3-phosphocholine involves large-scale chemical synthesis using similar methods as in laboratory synthesis but optimized for higher yields and purity. The process includes rigorous purification steps to ensure the final product meets the required standards for research and industrial applications .
Análisis De Reacciones Químicas
Types of Reactions: 1,2-Dierucoyl-sn-glycero-3-phosphocholine undergoes various chemical reactions, including hydrolysis, oxidation, and substitution reactions.
Common Reagents and Conditions:
Hydrolysis: This reaction can be catalyzed by acids or bases, leading to the breakdown of the phospholipid into its constituent fatty acids and glycerophosphocholine.
Major Products:
Hydrolysis Products: Erucic acid and glycerophosphocholine.
Oxidation Products: Peroxides and other oxidative derivatives of erucic acid.
Comparación Con Compuestos Similares
1,2-Dioleoyl-sn-glycero-3-phosphocholine: Contains oleic acid instead of erucic acid, resulting in different membrane properties.
1,2-Dipalmitoyl-sn-glycero-3-phosphocholine: Contains saturated fatty acids, leading to a more rigid membrane structure.
1,2-Distearoyl-sn-glycero-3-phosphocholine: Another saturated phospholipid with different physical properties compared to 1,2-Dierucoyl-sn-glycero-3-phosphocholine.
Uniqueness: 1,2-Dierucoyl-sn-glycero-3-phosphocholine is unique due to its long-chain unsaturated fatty acids, which confer distinct fluidity and permeability characteristics to lipid bilayers. This makes it particularly useful in studies involving membrane dynamics and in the formulation of flexible liposomal drug delivery systems .
Propiedades
IUPAC Name |
[(2R)-2,3-bis[[(Z)-docos-13-enoyl]oxy]propyl] 2-(trimethylazaniumyl)ethyl phosphate | |
---|---|---|
Source | PubChem | |
URL | https://pubchem.ncbi.nlm.nih.gov | |
Description | Data deposited in or computed by PubChem | |
InChI |
InChI=1S/C52H100NO8P/c1-6-8-10-12-14-16-18-20-22-24-26-28-30-32-34-36-38-40-42-44-51(54)58-48-50(49-60-62(56,57)59-47-46-53(3,4)5)61-52(55)45-43-41-39-37-35-33-31-29-27-25-23-21-19-17-15-13-11-9-7-2/h20-23,50H,6-19,24-49H2,1-5H3/b22-20-,23-21-/t50-/m1/s1 | |
Source | PubChem | |
URL | https://pubchem.ncbi.nlm.nih.gov | |
Description | Data deposited in or computed by PubChem | |
InChI Key |
SDEURMLKLAEUAY-JFSPZUDSSA-N | |
Source | PubChem | |
URL | https://pubchem.ncbi.nlm.nih.gov | |
Description | Data deposited in or computed by PubChem | |
Canonical SMILES |
CCCCCCCCC=CCCCCCCCCCCCC(=O)OCC(COP(=O)([O-])OCC[N+](C)(C)C)OC(=O)CCCCCCCCCCCC=CCCCCCCCC | |
Source | PubChem | |
URL | https://pubchem.ncbi.nlm.nih.gov | |
Description | Data deposited in or computed by PubChem | |
Isomeric SMILES |
CCCCCCCC/C=C\CCCCCCCCCCCC(=O)OC[C@H](COP(=O)([O-])OCC[N+](C)(C)C)OC(=O)CCCCCCCCCCC/C=C\CCCCCCCC | |
Source | PubChem | |
URL | https://pubchem.ncbi.nlm.nih.gov | |
Description | Data deposited in or computed by PubChem | |
Molecular Formula |
C52H100NO8P | |
Source | PubChem | |
URL | https://pubchem.ncbi.nlm.nih.gov | |
Description | Data deposited in or computed by PubChem | |
DSSTOX Substance ID |
DTXSID401345798 | |
Record name | 1,2-Dierucoyl-sn-glycero-3-phosphatidylcholine | |
Source | EPA DSSTox | |
URL | https://comptox.epa.gov/dashboard/DTXSID401345798 | |
Description | DSSTox provides a high quality public chemistry resource for supporting improved predictive toxicology. | |
Molecular Weight |
898.3 g/mol | |
Source | PubChem | |
URL | https://pubchem.ncbi.nlm.nih.gov | |
Description | Data deposited in or computed by PubChem | |
Physical Description |
Solid | |
Record name | PC(22:1(13Z)/22:1(13Z)) | |
Source | Human Metabolome Database (HMDB) | |
URL | http://www.hmdb.ca/metabolites/HMDB0008578 | |
Description | The Human Metabolome Database (HMDB) is a freely available electronic database containing detailed information about small molecule metabolites found in the human body. | |
Explanation | HMDB is offered to the public as a freely available resource. Use and re-distribution of the data, in whole or in part, for commercial purposes requires explicit permission of the authors and explicit acknowledgment of the source material (HMDB) and the original publication (see the HMDB citing page). We ask that users who download significant portions of the database cite the HMDB paper in any resulting publications. | |
CAS No. |
51779-95-4, 76420-81-0 | |
Record name | 1,2-Dierucoyl-sn-glycero-3-phosphocholine | |
Source | ChemIDplus | |
URL | https://pubchem.ncbi.nlm.nih.gov/substance/?source=chemidplus&sourceid=0051779954 | |
Description | ChemIDplus is a free, web search system that provides access to the structure and nomenclature authority files used for the identification of chemical substances cited in National Library of Medicine (NLM) databases, including the TOXNET system. | |
Record name | 1,2-Didocos-13-enoyl phoshatidylcholine | |
Source | ChemIDplus | |
URL | https://pubchem.ncbi.nlm.nih.gov/substance/?source=chemidplus&sourceid=0076420810 | |
Description | ChemIDplus is a free, web search system that provides access to the structure and nomenclature authority files used for the identification of chemical substances cited in National Library of Medicine (NLM) databases, including the TOXNET system. | |
Record name | 1,2-Dierucoyl-sn-glycero-3-phosphatidylcholine | |
Source | EPA DSSTox | |
URL | https://comptox.epa.gov/dashboard/DTXSID401345798 | |
Description | DSSTox provides a high quality public chemistry resource for supporting improved predictive toxicology. | |
Record name | 1,2-DIERUCOYL-SN-GLYCERO-3-PHOSPHOCHOLINE | |
Source | FDA Global Substance Registration System (GSRS) | |
URL | https://gsrs.ncats.nih.gov/ginas/app/beta/substances/1Z951826B6 | |
Description | The FDA Global Substance Registration System (GSRS) enables the efficient and accurate exchange of information on what substances are in regulated products. Instead of relying on names, which vary across regulatory domains, countries, and regions, the GSRS knowledge base makes it possible for substances to be defined by standardized, scientific descriptions. | |
Explanation | Unless otherwise noted, the contents of the FDA website (www.fda.gov), both text and graphics, are not copyrighted. They are in the public domain and may be republished, reprinted and otherwise used freely by anyone without the need to obtain permission from FDA. Credit to the U.S. Food and Drug Administration as the source is appreciated but not required. | |
Retrosynthesis Analysis
AI-Powered Synthesis Planning: Our tool employs the Template_relevance Pistachio, Template_relevance Bkms_metabolic, Template_relevance Pistachio_ringbreaker, Template_relevance Reaxys, Template_relevance Reaxys_biocatalysis model, leveraging a vast database of chemical reactions to predict feasible synthetic routes.
One-Step Synthesis Focus: Specifically designed for one-step synthesis, it provides concise and direct routes for your target compounds, streamlining the synthesis process.
Accurate Predictions: Utilizing the extensive PISTACHIO, BKMS_METABOLIC, PISTACHIO_RINGBREAKER, REAXYS, REAXYS_BIOCATALYSIS database, our tool offers high-accuracy predictions, reflecting the latest in chemical research and data.
Strategy Settings
Precursor scoring | Relevance Heuristic |
---|---|
Min. plausibility | 0.01 |
Model | Template_relevance |
Template Set | Pistachio/Bkms_metabolic/Pistachio_ringbreaker/Reaxys/Reaxys_biocatalysis |
Top-N result to add to graph | 6 |
Feasible Synthetic Routes
Q1: How does the acyl chain length of di22:1PC influence its interaction with membrane-active molecules like antimicrobial peptides?
A1: The long 22-carbon acyl chains of di22:1PC create a thicker bilayer compared to phospholipids with shorter chains, such as 1,2-dimyristoleoyl-sn-glycero-3-phosphocholine (DMPC) or 1,2-dioleoyl-sn-glycero-3-phosphocholine (DOPC) []. This difference in thickness significantly affects the activity of membrane-active molecules. For instance, synthetic ion channels with longer hydrophobic regions exhibit peak activity in di22:1PC liposomes, while shorter channels are more active in thinner DMPC membranes []. This suggests that matching the hydrophobic length of the active molecule to the bilayer thickness is crucial for optimal interaction. Similarly, the antimicrobial peptide melittin induces a greater thinning effect in di22:1PC bilayers compared to thinner bilayers, highlighting the role of bilayer thickness in peptide-membrane interactions [].
Q2: Can di22:1PC be used to study the impact of membrane thickness on the activity of pore-forming molecules?
A2: Yes, di22:1PC's ability to form thicker bilayers makes it a useful tool for investigating the influence of membrane thickness on pore formation. Research on the bacterial toxin colicin E1 demonstrates this. Colicin E1 forms small conductance channels in thinner membranes but switches to a novel large conductance state in di22:1PC bilayers []. This suggests that the thicker hydrophobic environment provided by di22:1PC can alter the conformation and properties of membrane-spanning protein regions, directly impacting their function.
Q3: How does di22:1PC contribute to understanding drug-membrane interactions?
A3: di22:1PC serves as a model membrane system to assess the bilayer-perturbing effects of drugs. Studies have shown that certain drug-like molecules can either enhance or suppress the function of gramicidin channels embedded in di22:1PC bilayers, while not affecting their activity in thinner DOPC bilayers []. This difference highlights the sensitivity of membrane proteins to drug-induced changes in bilayer properties, which can be more pronounced in thicker membranes like those formed by di22:1PC. By utilizing di22:1PC in in silico and in vitro assays, researchers can better predict the potential of drug candidates to perturb membrane structure and function, ultimately contributing to safer drug design [].
Descargo de responsabilidad e información sobre productos de investigación in vitro
Tenga en cuenta que todos los artículos e información de productos presentados en BenchChem están destinados únicamente con fines informativos. Los productos disponibles para la compra en BenchChem están diseñados específicamente para estudios in vitro, que se realizan fuera de organismos vivos. Los estudios in vitro, derivados del término latino "in vidrio", involucran experimentos realizados en entornos de laboratorio controlados utilizando células o tejidos. Es importante tener en cuenta que estos productos no se clasifican como medicamentos y no han recibido la aprobación de la FDA para la prevención, tratamiento o cura de ninguna condición médica, dolencia o enfermedad. Debemos enfatizar que cualquier forma de introducción corporal de estos productos en humanos o animales está estrictamente prohibida por ley. Es esencial adherirse a estas pautas para garantizar el cumplimiento de los estándares legales y éticos en la investigación y experimentación.