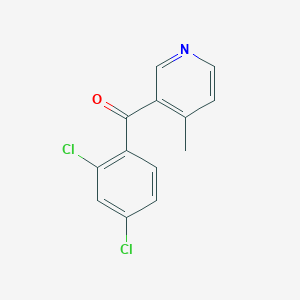
3-(2,4-Dichlorobenzoyl)-4-methylpyridine
Descripción general
Descripción
Aplicaciones Científicas De Investigación
Hydrogen Bonded Supramolecular Structures
The compound 3-(2,4-Dichlorobenzoyl)-4-methylpyridine and its derivatives show remarkable applications in the formation of hydrogen-bonded supramolecular structures. Studies indicate that various molecular salts formed with this compound exhibit organic salt characteristics, with proton transfer to the pyridine nitrogen of the 2-amino-4-methylpyridine moiety. The structures formed adopt supramolecular [R22(8)] heterosynthons, connected via N–H···O hydrogen bonds, forming supramolecular chains in most of the salts. These structures are stabilized by extensive classical hydrogen bonds and other noncovalent interactions, displaying 1D–3D framework structures due to the synergistic effect of various non-covalent interactions (Khalib et al., 2014).
Crystal Structure and Synthesis Applications
This compound has been utilized in the synthesis of complex compounds. A particular instance involves the synthesis of a novel compound through specific reactions, resulting in a dimer linked by intermolecular hydrogen bonds, as observed in the crystal structure determined by X-ray diffraction. The structure exhibits unique features such as hydrogen bonding between specific nitrogen and oxygen atoms, indicating the compound's relevance in synthesis and structure elucidation (Xia, 2001).
Separation and Purification in Medicinal and Pesticide Intermediates
Compounds related to this compound, like 2-Chloro-5-trichloromethylpyridine, are essential intermediates in various medicines and pesticides. The methodologies used in the separation and purification of these compounds, such as extraction, distillation, and column chromatography, highlight the compound's significance in the pharmaceutical and agricultural sectors. The high purity levels achieved, surpassing 99%, underline the efficiency of these methods and the importance of these compounds in producing high-quality products (Li, 2005).
Mecanismo De Acción
Target of Action
The primary target of 3-(2,4-Dichlorobenzoyl)-4-methylpyridine is the enzyme Phytoene Desaturase (PD) . This enzyme plays a crucial role in the carotenogenic pathway, where it catalyzes the hydrogen abstraction step at the first C40 precursor of β-carotene .
Mode of Action
This compound inhibits the Phytoene Desaturase enzyme This inhibition disrupts the normal function of the enzyme, leading to changes in the carotenogenic pathway
Biochemical Pathways
The inhibition of Phytoene Desaturase by this compound affects the carotenogenic pathway . This pathway is responsible for the production of carotenoids, which are important for plant growth and development. Disruption of this pathway can lead to a variety of downstream effects, including growth inhibition and bleaching.
Result of Action
The inhibition of Phytoene Desaturase by this compound leads to disruption of the carotenogenic pathway . This can result in a variety of molecular and cellular effects, including growth inhibition and bleaching. The exact effects would depend on the specific organism and the extent of enzyme inhibition.
Safety and Hazards
Direcciones Futuras
Propiedades
IUPAC Name |
(2,4-dichlorophenyl)-(4-methylpyridin-3-yl)methanone | |
---|---|---|
Source | PubChem | |
URL | https://pubchem.ncbi.nlm.nih.gov | |
Description | Data deposited in or computed by PubChem | |
InChI |
InChI=1S/C13H9Cl2NO/c1-8-4-5-16-7-11(8)13(17)10-3-2-9(14)6-12(10)15/h2-7H,1H3 | |
Source | PubChem | |
URL | https://pubchem.ncbi.nlm.nih.gov | |
Description | Data deposited in or computed by PubChem | |
InChI Key |
ZIIIHEGBCGICQI-UHFFFAOYSA-N | |
Source | PubChem | |
URL | https://pubchem.ncbi.nlm.nih.gov | |
Description | Data deposited in or computed by PubChem | |
Canonical SMILES |
CC1=C(C=NC=C1)C(=O)C2=C(C=C(C=C2)Cl)Cl | |
Source | PubChem | |
URL | https://pubchem.ncbi.nlm.nih.gov | |
Description | Data deposited in or computed by PubChem | |
Molecular Formula |
C13H9Cl2NO | |
Source | PubChem | |
URL | https://pubchem.ncbi.nlm.nih.gov | |
Description | Data deposited in or computed by PubChem | |
Molecular Weight |
266.12 g/mol | |
Source | PubChem | |
URL | https://pubchem.ncbi.nlm.nih.gov | |
Description | Data deposited in or computed by PubChem | |
Retrosynthesis Analysis
AI-Powered Synthesis Planning: Our tool employs the Template_relevance Pistachio, Template_relevance Bkms_metabolic, Template_relevance Pistachio_ringbreaker, Template_relevance Reaxys, Template_relevance Reaxys_biocatalysis model, leveraging a vast database of chemical reactions to predict feasible synthetic routes.
One-Step Synthesis Focus: Specifically designed for one-step synthesis, it provides concise and direct routes for your target compounds, streamlining the synthesis process.
Accurate Predictions: Utilizing the extensive PISTACHIO, BKMS_METABOLIC, PISTACHIO_RINGBREAKER, REAXYS, REAXYS_BIOCATALYSIS database, our tool offers high-accuracy predictions, reflecting the latest in chemical research and data.
Strategy Settings
Precursor scoring | Relevance Heuristic |
---|---|
Min. plausibility | 0.01 |
Model | Template_relevance |
Template Set | Pistachio/Bkms_metabolic/Pistachio_ringbreaker/Reaxys/Reaxys_biocatalysis |
Top-N result to add to graph | 6 |
Feasible Synthetic Routes
Descargo de responsabilidad e información sobre productos de investigación in vitro
Tenga en cuenta que todos los artículos e información de productos presentados en BenchChem están destinados únicamente con fines informativos. Los productos disponibles para la compra en BenchChem están diseñados específicamente para estudios in vitro, que se realizan fuera de organismos vivos. Los estudios in vitro, derivados del término latino "in vidrio", involucran experimentos realizados en entornos de laboratorio controlados utilizando células o tejidos. Es importante tener en cuenta que estos productos no se clasifican como medicamentos y no han recibido la aprobación de la FDA para la prevención, tratamiento o cura de ninguna condición médica, dolencia o enfermedad. Debemos enfatizar que cualquier forma de introducción corporal de estos productos en humanos o animales está estrictamente prohibida por ley. Es esencial adherirse a estas pautas para garantizar el cumplimiento de los estándares legales y éticos en la investigación y experimentación.