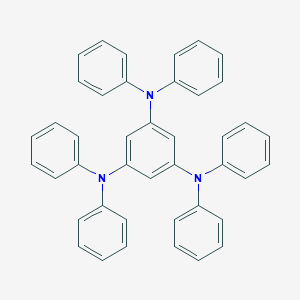
1,3,5-Tris(diphenylamino)benzene
Descripción general
Descripción
1,3,5-Tris(diphenylamino)benzene is a useful research compound. Its molecular formula is C42H33N3 and its molecular weight is 579.7 g/mol. The purity is usually 95%.
BenchChem offers high-quality this compound suitable for many research applications. Different packaging options are available to accommodate customers' requirements. Please inquire for more information about this compound including the price, delivery time, and more detailed information at info@benchchem.com.
Actividad Biológica
1,3,5-Tris(diphenylamino)benzene (TPAB) is a compound of significant interest due to its unique structural properties and potential applications in various fields, including organic electronics and biomedicine. This article reviews the biological activity of TPAB, synthesizing findings from diverse studies to provide a comprehensive overview.
Chemical Structure and Properties
This compound features a central benzene ring with three diphenylamino substituents. This configuration contributes to its electronic properties, making it a candidate for applications as a hole transport material in organic light-emitting diodes (OLEDs) and perovskite solar cells . The compound exhibits strong photoluminescence and has been studied for its interactions with various biological systems.
Antioxidant Properties
Recent studies have highlighted the antioxidant capabilities of TPAB. It has been shown to scavenge free radicals effectively, which may contribute to its protective effects against oxidative stress in cells. This property is particularly relevant in the context of neuroprotection and cancer therapy .
Cytotoxicity and Anticancer Activity
TPAB has demonstrated cytotoxic effects against several cancer cell lines. For instance, research indicates that TPAB can induce apoptosis in human leukemia cells through the activation of caspase pathways. The compound's ability to modulate cellular signaling pathways makes it a promising candidate for further investigation as an anticancer agent .
Case Studies
- Neuroprotective Effects : A study investigated the neuroprotective effects of TPAB in models of neurodegenerative diseases. The results indicated that TPAB reduced neuronal cell death induced by oxidative stress, suggesting its potential use in treating conditions like Alzheimer's disease .
- Anticancer Efficacy : In vitro studies on human breast cancer cells revealed that TPAB inhibited cell proliferation and induced cell cycle arrest at the G2/M phase. These findings support the compound's potential as a therapeutic agent in breast cancer treatment .
The biological activity of TPAB is attributed to several mechanisms:
- Radical Scavenging : TPAB's structure allows it to effectively neutralize reactive oxygen species (ROS), thereby mitigating oxidative damage to cells .
- Apoptosis Induction : The compound activates intrinsic apoptotic pathways, leading to increased caspase activity and subsequent cell death in cancerous cells .
- Modulation of Signaling Pathways : TPAB influences various signaling pathways involved in cell survival and proliferation, enhancing its anticancer properties .
Data Table: Summary of Biological Activities
Future Directions
Given the promising biological activities exhibited by this compound, future research should focus on:
- In Vivo Studies : To evaluate the therapeutic potential and safety profile of TPAB in animal models.
- Mechanistic Studies : Further elucidation of the molecular mechanisms underlying its biological effects.
- Formulation Development : Exploring formulations that enhance bioavailability and target delivery for clinical applications.
Aplicaciones Científicas De Investigación
Hole Transport Materials (HTMs)
TDAB is primarily utilized as a hole transport material in organic light-emitting diodes (OLEDs) and perovskite solar cells. Its high hole mobility facilitates efficient charge transport, which is crucial for the performance of these devices. Research indicates that derivatives of TDAB exhibit promising characteristics for enhancing the efficiency of perovskite solar cells .
- Performance Metrics :
- High hole mobility
- Low electron affinity
- Stability under operational conditions
Organic Photodetectors
In photodetector applications, TDAB's ability to act as an electron-blocking layer enhances device performance by preventing recombination losses. This property is vital for improving the sensitivity and response time of organic photodetectors .
Triazine-Based Conjugated Microporous Polymers
A study explored the incorporation of TDAB into triazine-based conjugated microporous polymers (TCMPs), which demonstrated exceptional iodine capture capabilities and fluorescence sensing for nitrophenol detection. The TCMPs exhibited a high BET surface area and significant adsorption capacities .
Property | Value |
---|---|
BET Surface Area | >512 m²/g |
Iodine Adsorption | 3.13 g/g |
Detection Sensitivity | High |
Synthesis of Geometrically Modified TDAB Derivatives
Research on geometrically modified TDAB derivatives has shown that altering the molecular structure can lead to enhanced photoluminescent properties. These modifications allow for tailored applications in optoelectronics, where specific emission characteristics are required for device functionality .
Propiedades
IUPAC Name |
1-N,1-N,3-N,3-N,5-N,5-N-hexakis-phenylbenzene-1,3,5-triamine | |
---|---|---|
Source | PubChem | |
URL | https://pubchem.ncbi.nlm.nih.gov | |
Description | Data deposited in or computed by PubChem | |
InChI |
InChI=1S/C42H33N3/c1-7-19-34(20-8-1)43(35-21-9-2-10-22-35)40-31-41(44(36-23-11-3-12-24-36)37-25-13-4-14-26-37)33-42(32-40)45(38-27-15-5-16-28-38)39-29-17-6-18-30-39/h1-33H | |
Source | PubChem | |
URL | https://pubchem.ncbi.nlm.nih.gov | |
Description | Data deposited in or computed by PubChem | |
InChI Key |
DPFGGYIWNDCEJM-UHFFFAOYSA-N | |
Source | PubChem | |
URL | https://pubchem.ncbi.nlm.nih.gov | |
Description | Data deposited in or computed by PubChem | |
Canonical SMILES |
C1=CC=C(C=C1)N(C2=CC=CC=C2)C3=CC(=CC(=C3)N(C4=CC=CC=C4)C5=CC=CC=C5)N(C6=CC=CC=C6)C7=CC=CC=C7 | |
Source | PubChem | |
URL | https://pubchem.ncbi.nlm.nih.gov | |
Description | Data deposited in or computed by PubChem | |
Molecular Formula |
C42H33N3 | |
Source | PubChem | |
URL | https://pubchem.ncbi.nlm.nih.gov | |
Description | Data deposited in or computed by PubChem | |
DSSTOX Substance ID |
DTXSID30566826 | |
Record name | N~1~,N~1~,N~3~,N~3~,N~5~,N~5~-Hexaphenylbenzene-1,3,5-triamine | |
Source | EPA DSSTox | |
URL | https://comptox.epa.gov/dashboard/DTXSID30566826 | |
Description | DSSTox provides a high quality public chemistry resource for supporting improved predictive toxicology. | |
Molecular Weight |
579.7 g/mol | |
Source | PubChem | |
URL | https://pubchem.ncbi.nlm.nih.gov | |
Description | Data deposited in or computed by PubChem | |
CAS No. |
126717-23-5 | |
Record name | N~1~,N~1~,N~3~,N~3~,N~5~,N~5~-Hexaphenylbenzene-1,3,5-triamine | |
Source | EPA DSSTox | |
URL | https://comptox.epa.gov/dashboard/DTXSID30566826 | |
Description | DSSTox provides a high quality public chemistry resource for supporting improved predictive toxicology. | |
Retrosynthesis Analysis
AI-Powered Synthesis Planning: Our tool employs the Template_relevance Pistachio, Template_relevance Bkms_metabolic, Template_relevance Pistachio_ringbreaker, Template_relevance Reaxys, Template_relevance Reaxys_biocatalysis model, leveraging a vast database of chemical reactions to predict feasible synthetic routes.
One-Step Synthesis Focus: Specifically designed for one-step synthesis, it provides concise and direct routes for your target compounds, streamlining the synthesis process.
Accurate Predictions: Utilizing the extensive PISTACHIO, BKMS_METABOLIC, PISTACHIO_RINGBREAKER, REAXYS, REAXYS_BIOCATALYSIS database, our tool offers high-accuracy predictions, reflecting the latest in chemical research and data.
Strategy Settings
Precursor scoring | Relevance Heuristic |
---|---|
Min. plausibility | 0.01 |
Model | Template_relevance |
Template Set | Pistachio/Bkms_metabolic/Pistachio_ringbreaker/Reaxys/Reaxys_biocatalysis |
Top-N result to add to graph | 6 |
Feasible Synthetic Routes
ANone: 1,3,5-Tris(diphenylamino)benzene has a molecular formula of C42H33N3 and a molecular weight of 579.72 g/mol.
A: Methyl-substituted derivatives of TDAB readily form stable amorphous glasses, a characteristic crucial for various applications. [, ] These glasses exhibit glass-transition temperatures around 50 °C. []
A: Research indicates a significant influence of the methyl substituent on the formation and properties of the glassy state in TDAB derivatives. []
A: Studies on compounds like 5, 5″‐Bis{4‐[bis(4‐methylphenyl)amino]phenyl}2, 2′:5′, 2″‐terthiophene, which incorporate oligothiophene units into a TDAB-like structure, explore their potential as amorphous molecular materials for applications such as organic electroluminescent devices. []
A: Yes, molecular orbital studies have explored the electronic structure of TDAB, particularly its cationic triradical state. [] Density functional theory (DFT) and time-dependent DFT (TD-DFT) calculations have been employed to investigate the potential of TDAB derivatives as hole transport materials in perovskite solar cells. []
A: TDAB undergoes photocyclization reactions, both in the presence and absence of oxygen. [, ] This process leads to the formation of N-phenyl-2,4-bis(diphenylamino)carbazole. The reaction mechanism differs depending on the presence or absence of oxygen, involving the excited triplet state of TDAB and potentially a dihydrocarbazole radical cation intermediate. []
A: The electrochemical oxidation of TDAB has been explored for its potential in generating polyradical materials. [] This area holds interest due to the unique electronic and magnetic properties of polyradicals.
A: Yes, methyl-substituted derivatives of TDAB, specifically 1,3,5-tris(2-methylphenylphenylamino)benzene and 1,3,5-tris(4-methylphenylphenylamino)benzene, exhibit polymorphism. [] This means they can exist in different crystal structures, impacting their physical properties and potential applications.
A: Common analytical techniques used to characterize TDAB include differential scanning calorimetry (DSC) to study its thermal transitions, X-ray diffraction (XRD) to determine its crystal structure, and various spectroscopic methods such as electron spin resonance (ESR) to investigate its electronic structure. [, , , ]
Descargo de responsabilidad e información sobre productos de investigación in vitro
Tenga en cuenta que todos los artículos e información de productos presentados en BenchChem están destinados únicamente con fines informativos. Los productos disponibles para la compra en BenchChem están diseñados específicamente para estudios in vitro, que se realizan fuera de organismos vivos. Los estudios in vitro, derivados del término latino "in vidrio", involucran experimentos realizados en entornos de laboratorio controlados utilizando células o tejidos. Es importante tener en cuenta que estos productos no se clasifican como medicamentos y no han recibido la aprobación de la FDA para la prevención, tratamiento o cura de ninguna condición médica, dolencia o enfermedad. Debemos enfatizar que cualquier forma de introducción corporal de estos productos en humanos o animales está estrictamente prohibida por ley. Es esencial adherirse a estas pautas para garantizar el cumplimiento de los estándares legales y éticos en la investigación y experimentación.