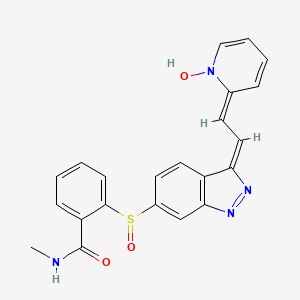
Axitinib metabolite M9
Descripción general
Descripción
Axitinib metabolite M9 is a derivative of axitinib, a potent and selective second-generation inhibitor of vascular endothelial growth factor receptors 1, 2, and 3. Axitinib is primarily used in the treatment of advanced renal cell carcinoma. The metabolite M9 is one of the several metabolites formed during the biotransformation of axitinib in the human body .
Métodos De Preparación
The preparation of axitinib metabolite M9 involves the metabolic processes in the human liver, primarily mediated by cytochrome P450 enzymes, particularly CYP3A4/5, with minor contributions from CYP2C19 and CYP1A2 . Industrial production methods for axitinib itself involve complex synthetic routes, including the formation of various intermediates and the use of specific reagents and conditions to achieve the desired chemical structure .
Análisis De Reacciones Químicas
Axitinib metabolite M9 undergoes several types of chemical reactions, including oxidation and glucuronidation. The primary enzymes involved in these reactions are cytochrome P450 (CYP) enzymes and uridine diphosphate glucuronosyltransferase (UGT) enzymes . Common reagents used in these reactions include NADPH and UDPGA. The major products formed from these reactions are axitinib sulfoxide and axitinib N-glucuronide .
Aplicaciones Científicas De Investigación
Axitinib metabolite M9 has significant scientific research applications, particularly in the fields of pharmacokinetics and drug metabolism. It is used to study the metabolic pathways and clearance mechanisms of axitinib in the human body . Additionally, it helps in understanding the pharmacological and toxicological profiles of axitinib, contributing to the development of more effective and safer therapeutic strategies .
Mecanismo De Acción
The mechanism of action of axitinib metabolite M9 involves the inhibition of vascular endothelial growth factor receptors (VEGFRs) 1, 2, and 3. By blocking these receptors, the metabolite inhibits angiogenesis, tumor growth, and metastasis . This action is crucial in the treatment of advanced renal cell carcinoma, as it helps to reduce the blood supply to the tumor, thereby inhibiting its growth and spread .
Comparación Con Compuestos Similares
Axitinib metabolite M9 can be compared with other similar compounds, such as sorafenib, sunitinib, and pazopanib, which are also tyrosine kinase inhibitors used in the treatment of renal cell carcinoma . While all these compounds target VEGFRs, axitinib and its metabolites are known for their higher potency and selectivity . This uniqueness makes axitinib and its metabolites, including M9, particularly effective in inhibiting angiogenesis and tumor growth .
Q & A
Basic Research Questions
Q. How is Axitinib metabolite M9 identified and quantified in pharmacokinetic (PK) studies, and what methodological considerations ensure accuracy?
- Answer : Metabolite M9 is typically quantified using liquid chromatography-tandem mass spectrometry (LC-MS/MS) due to its high sensitivity and specificity for low-concentration analytes. In PK studies, blood samples are collected at predefined intervals post-dosing, and plasma concentrations of M9 are measured against validated calibration curves. Critical considerations include:
- Matrix effects : Use of stable isotope-labeled internal standards to correct for ion suppression/enhancement .
- Metabolic stability : Assessment of M9 stability in plasma under varying storage conditions to prevent degradation .
- Cross-validation : Harmonization of analytical methods across multi-site trials to minimize inter-lab variability .
Q. What experimental designs are employed to assess M9's formation kinetics and metabolic pathways in preclinical models?
- Answer : Preclinical studies often use hepatocyte incubations or liver microsomes from multiple species (e.g., human, rat, dog) to evaluate interspecies metabolic differences. Key steps include:
- Reaction phenotyping : Inhibition assays with CYP-specific inhibitors (e.g., CYP3A4 for Axitinib) to identify enzymes responsible for M9 formation .
- Time-course analyses : Sampling at multiple time points to derive formation/elimination half-lives and assess auto-inhibition or enzyme saturation .
Advanced Research Questions
Q. How does M9 contribute to observed drug-drug interaction (DDI) risks when Axitinib is combined with cisplatin/capecitabine?
- Answer : Co-administration of Axitinib with cisplatin/capecitabine in Phase I trials revealed a ~30% increase in Axitinib exposure, potentially due to M9-mediated inhibition of efflux transporters (e.g., P-glycoprotein). Methodological approaches to resolve this include:
- Transporter assays : In vitro models (e.g., Caco-2 cells) to test M9’s affinity for transporters involved in Axitinib disposition .
- Physiologically based pharmacokinetic (PBPK) modeling : Integration of metabolite-specific parameters to predict DDIs in virtual populations .
- Contradiction note : While cisplatin did not alter Axitinib PK, capecitabine/5-FU exposure decreased, suggesting complex interplay between M9 and fluoropyrimidine metabolism .
Q. What strategies address variability in M9 plasma exposure across patient populations, and how does this impact dose optimization?
- Answer : Population pharmacokinetic (PopPK) modeling is used to identify covariates (e.g., CYP3A4 polymorphisms, renal/hepatic function) influencing M9 variability. For example:
- Dose titration : In Phase II trials, patients with low Axitinib/M9 exposure underwent dose escalation (up to 10 mg BID) if tolerability criteria (e.g., blood pressure, adverse events) were met .
- Covariate analysis : Retrospective genotyping of trial cohorts to link polymorphisms in CYP3A4/5 or UGT1A1 to M9 exposure .
Q. How can in vitro-in vivo extrapolation (IVIVE) models improve safety assessments of M9, particularly for irreversible toxicity risks?
- Answer : IVIVE integrates in vitro toxicity data (e.g., mitochondrial ROS inhibition in hepatic stellate cells) with human PK parameters to predict M9’s safety margin. Key steps:
- Reactive metabolite screening : Glutathione-trapping assays to detect M9-derived electrophilic intermediates .
- Toxicity thresholds : Benchmark dose (BMD) modeling using preclinical data from liver fibrosis models, where M9 showed mitochondrial protective effects at IC₅₀ < 100 nM .
Q. Contradiction Analysis and Mitigation
Q. How should researchers resolve conflicting data on M9’s role in Axitinib efficacy between monotherapy and combination regimens?
- Answer : In monotherapy (e.g., renal cell carcinoma), M9’s contribution to efficacy is inferred from PopPK-pharmacodynamic (PD) models showing exposure-response relationships for progression-free survival . In combination therapies (e.g., pembrolizumab + Axitinib), randomized trials use stratified randomization by metabolic phenotype (extensive vs. poor metabolizers) to isolate M9’s immunomodulatory effects .
- Mitigation strategy : Adaptive trial designs with interim PK/PD analyses to adjust for metabolite-specific confounding .
Q. Methodological Resources
Propiedades
IUPAC Name |
2-[(3E)-3-[(2E)-2-(1-hydroxypyridin-2-ylidene)ethylidene]indazol-6-yl]sulfinyl-N-methylbenzamide | |
---|---|---|
Source | PubChem | |
URL | https://pubchem.ncbi.nlm.nih.gov | |
Description | Data deposited in or computed by PubChem | |
InChI |
InChI=1S/C22H18N4O3S/c1-23-22(27)18-7-2-3-8-21(18)30(29)16-10-11-17-19(24-25-20(17)14-16)12-9-15-6-4-5-13-26(15)28/h2-14,28H,1H3,(H,23,27)/b15-9+,19-12+ | |
Source | PubChem | |
URL | https://pubchem.ncbi.nlm.nih.gov | |
Description | Data deposited in or computed by PubChem | |
InChI Key |
MESVCUNKPBREDN-UUAZFVJBSA-N | |
Source | PubChem | |
URL | https://pubchem.ncbi.nlm.nih.gov | |
Description | Data deposited in or computed by PubChem | |
Canonical SMILES |
CNC(=O)C1=CC=CC=C1S(=O)C2=CC3=C(C=C2)C(=CC=C4C=CC=CN4O)N=N3 | |
Source | PubChem | |
URL | https://pubchem.ncbi.nlm.nih.gov | |
Description | Data deposited in or computed by PubChem | |
Isomeric SMILES |
CNC(=O)C1=CC=CC=C1S(=O)C2=CC3=C(C=C2)/C(=C\C=C\4/C=CC=CN4O)/N=N3 | |
Source | PubChem | |
URL | https://pubchem.ncbi.nlm.nih.gov | |
Description | Data deposited in or computed by PubChem | |
Molecular Formula |
C22H18N4O3S | |
Source | PubChem | |
URL | https://pubchem.ncbi.nlm.nih.gov | |
Description | Data deposited in or computed by PubChem | |
Molecular Weight |
418.5 g/mol | |
Source | PubChem | |
URL | https://pubchem.ncbi.nlm.nih.gov | |
Description | Data deposited in or computed by PubChem | |
CAS No. |
1348044-24-5 | |
Record name | Axitinib sulfoxide pyridine-N-oxide | |
Source | ChemIDplus | |
URL | https://pubchem.ncbi.nlm.nih.gov/substance/?source=chemidplus&sourceid=1348044245 | |
Description | ChemIDplus is a free, web search system that provides access to the structure and nomenclature authority files used for the identification of chemical substances cited in National Library of Medicine (NLM) databases, including the TOXNET system. | |
Record name | AXITINIB SULFOXIDE PYRIDINE-N-OXIDE | |
Source | FDA Global Substance Registration System (GSRS) | |
URL | https://gsrs.ncats.nih.gov/ginas/app/beta/substances/6ID0Q0B0IK | |
Description | The FDA Global Substance Registration System (GSRS) enables the efficient and accurate exchange of information on what substances are in regulated products. Instead of relying on names, which vary across regulatory domains, countries, and regions, the GSRS knowledge base makes it possible for substances to be defined by standardized, scientific descriptions. | |
Explanation | Unless otherwise noted, the contents of the FDA website (www.fda.gov), both text and graphics, are not copyrighted. They are in the public domain and may be republished, reprinted and otherwise used freely by anyone without the need to obtain permission from FDA. Credit to the U.S. Food and Drug Administration as the source is appreciated but not required. | |
Retrosynthesis Analysis
AI-Powered Synthesis Planning: Our tool employs the Template_relevance Pistachio, Template_relevance Bkms_metabolic, Template_relevance Pistachio_ringbreaker, Template_relevance Reaxys, Template_relevance Reaxys_biocatalysis model, leveraging a vast database of chemical reactions to predict feasible synthetic routes.
One-Step Synthesis Focus: Specifically designed for one-step synthesis, it provides concise and direct routes for your target compounds, streamlining the synthesis process.
Accurate Predictions: Utilizing the extensive PISTACHIO, BKMS_METABOLIC, PISTACHIO_RINGBREAKER, REAXYS, REAXYS_BIOCATALYSIS database, our tool offers high-accuracy predictions, reflecting the latest in chemical research and data.
Strategy Settings
Precursor scoring | Relevance Heuristic |
---|---|
Min. plausibility | 0.01 |
Model | Template_relevance |
Template Set | Pistachio/Bkms_metabolic/Pistachio_ringbreaker/Reaxys/Reaxys_biocatalysis |
Top-N result to add to graph | 6 |
Feasible Synthetic Routes
Descargo de responsabilidad e información sobre productos de investigación in vitro
Tenga en cuenta que todos los artículos e información de productos presentados en BenchChem están destinados únicamente con fines informativos. Los productos disponibles para la compra en BenchChem están diseñados específicamente para estudios in vitro, que se realizan fuera de organismos vivos. Los estudios in vitro, derivados del término latino "in vidrio", involucran experimentos realizados en entornos de laboratorio controlados utilizando células o tejidos. Es importante tener en cuenta que estos productos no se clasifican como medicamentos y no han recibido la aprobación de la FDA para la prevención, tratamiento o cura de ninguna condición médica, dolencia o enfermedad. Debemos enfatizar que cualquier forma de introducción corporal de estos productos en humanos o animales está estrictamente prohibida por ley. Es esencial adherirse a estas pautas para garantizar el cumplimiento de los estándares legales y éticos en la investigación y experimentación.