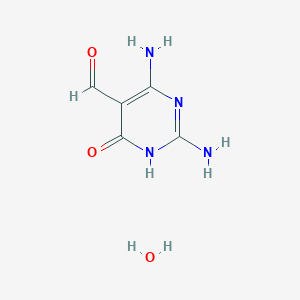
2,4-Diamino-6-hydroxypyrimidine-5-carbaldehyde hydrate
Descripción general
Descripción
2,4-Diamino-6-hydroxypyrimidine-5-carbaldehyde hydrate is a chemical compound with the molecular formula C5H6N4O2.H2O . It is a solid substance and its IUPAC name is 2,4-diamino-6-hydroxy-5-pyrimidinecarbaldehyde hydrate .
Molecular Structure Analysis
The molecular weight of this compound is 172.14 . The InChI code for this compound is 1S/C5H6N4O2.H2O/c6-3-2(1-10)4(11)9-5(7)8-3;/h1H,(H5,6,7,8,9,11);1H2 .Physical and Chemical Properties Analysis
The physical and chemical properties of this compound include a density of 1.9±0.1 g/cm3, a boiling point of 449.8±55.0 °C at 760 mmHg, and a vapor pressure of 0.0±1.1 mmHg at 25°C . The compound has a molar refractivity of 34.7±0.5 cm3, a polar surface area of 111 Å2, a polarizability of 13.7±0.5 10-24 cm3, and a molar volume of 82.0±7.0 cm3 .Aplicaciones Científicas De Investigación
Food Safety Evaluation : The substance has been evaluated for safety in food contact materials, particularly in rigid poly(vinyl chloride) (PVC), where it poses no safety concern for consumers under specified conditions (Flavourings, 2009).
Corrosion Inhibition : This compound serves as an effective corrosion inhibitor for mild steel in acidic media, with the inhibition effectiveness directly related to the concentration of the compound (Reşit Yıldız, 2018).
Chemical Synthesis : It is used in the synthesis of various chemical structures, including substituted tetrahydropyrimido[4,5-c][2,7]naphthyridines, a class of tricyclic 5-deaza nonclassical folates (A. Gangjee & K. Ohemeng, 1985).
Microbial Growth Inhibition : The compound and its derivatives have been studied for their inhibitory action on the growth of various microorganisms, such as Streptococcus faecalis and Escherichia coli (D. Roy, S. Ghosh, & B. C. Guha, 1961).
Antiviral Activity : Certain derivatives of this compound have shown antiviral activity, particularly against retroviruses in cell culture (D. Hocková et al., 2003).
Green Chemistry : Its derivatives have been synthesized under solvent-free conditions, highlighting an environmentally friendly approach to chemical synthesis (Liangce Rong et al., 2010).
Spectroscopy and Quantum Chemistry : The compound has been analyzed using FT-IR and FT-Raman spectroscopy, and quantum chemical calculations, providing insights into its molecular structure and properties (M. Subramanian, P. M. Anbarasan, & S. Manimegalai, 2009).
Pharmaceutical Chemistry : It has been involved in the synthesis of potential antineoplastic agents, demonstrating its relevance in drug discovery (H. Garg & R. Sharma, 1970).
Safety and Hazards
The safety information for 2,4-Diamino-6-hydroxypyrimidine-5-carbaldehyde hydrate includes hazard statements H302-H315-H319-H335, which indicate that the compound is harmful if swallowed, causes skin irritation, causes serious eye irritation, and may cause respiratory irritation . The precautionary statements include P261-P280-P301+P312-P302+P352-P305+P351+P338, which advise avoiding breathing dust/fume/gas/mist/vapors/spray, wearing protective gloves/protective clothing/eye protection/face protection, calling a POISON CENTER or doctor/physician if feeling unwell, washing with plenty of soap and water, and rinsing cautiously with water for several minutes .
Propiedades
IUPAC Name |
2,4-diamino-6-oxo-1H-pyrimidine-5-carbaldehyde;hydrate | |
---|---|---|
Source | PubChem | |
URL | https://pubchem.ncbi.nlm.nih.gov | |
Description | Data deposited in or computed by PubChem | |
InChI |
InChI=1S/C5H6N4O2.H2O/c6-3-2(1-10)4(11)9-5(7)8-3;/h1H,(H5,6,7,8,9,11);1H2 | |
Source | PubChem | |
URL | https://pubchem.ncbi.nlm.nih.gov | |
Description | Data deposited in or computed by PubChem | |
InChI Key |
NKFDKUYIGQNBPW-UHFFFAOYSA-N | |
Source | PubChem | |
URL | https://pubchem.ncbi.nlm.nih.gov | |
Description | Data deposited in or computed by PubChem | |
Canonical SMILES |
C(=O)C1=C(N=C(NC1=O)N)N.O | |
Source | PubChem | |
URL | https://pubchem.ncbi.nlm.nih.gov | |
Description | Data deposited in or computed by PubChem | |
Molecular Formula |
C5H8N4O3 | |
Source | PubChem | |
URL | https://pubchem.ncbi.nlm.nih.gov | |
Description | Data deposited in or computed by PubChem | |
Molecular Weight |
172.14 g/mol | |
Source | PubChem | |
URL | https://pubchem.ncbi.nlm.nih.gov | |
Description | Data deposited in or computed by PubChem | |
Retrosynthesis Analysis
AI-Powered Synthesis Planning: Our tool employs the Template_relevance Pistachio, Template_relevance Bkms_metabolic, Template_relevance Pistachio_ringbreaker, Template_relevance Reaxys, Template_relevance Reaxys_biocatalysis model, leveraging a vast database of chemical reactions to predict feasible synthetic routes.
One-Step Synthesis Focus: Specifically designed for one-step synthesis, it provides concise and direct routes for your target compounds, streamlining the synthesis process.
Accurate Predictions: Utilizing the extensive PISTACHIO, BKMS_METABOLIC, PISTACHIO_RINGBREAKER, REAXYS, REAXYS_BIOCATALYSIS database, our tool offers high-accuracy predictions, reflecting the latest in chemical research and data.
Strategy Settings
Precursor scoring | Relevance Heuristic |
---|---|
Min. plausibility | 0.01 |
Model | Template_relevance |
Template Set | Pistachio/Bkms_metabolic/Pistachio_ringbreaker/Reaxys/Reaxys_biocatalysis |
Top-N result to add to graph | 6 |
Feasible Synthetic Routes
Descargo de responsabilidad e información sobre productos de investigación in vitro
Tenga en cuenta que todos los artículos e información de productos presentados en BenchChem están destinados únicamente con fines informativos. Los productos disponibles para la compra en BenchChem están diseñados específicamente para estudios in vitro, que se realizan fuera de organismos vivos. Los estudios in vitro, derivados del término latino "in vidrio", involucran experimentos realizados en entornos de laboratorio controlados utilizando células o tejidos. Es importante tener en cuenta que estos productos no se clasifican como medicamentos y no han recibido la aprobación de la FDA para la prevención, tratamiento o cura de ninguna condición médica, dolencia o enfermedad. Debemos enfatizar que cualquier forma de introducción corporal de estos productos en humanos o animales está estrictamente prohibida por ley. Es esencial adherirse a estas pautas para garantizar el cumplimiento de los estándares legales y éticos en la investigación y experimentación.