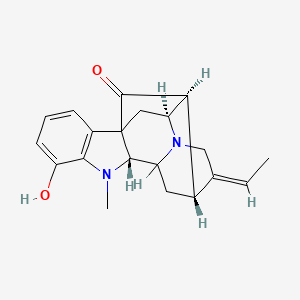
Mitoridine
Descripción general
Descripción
Mitoridine is a compound known for its vasopressor and antihypotensive properties, primarily used to treat conditions such as orthostatic hypotension. It works by increasing blood pressure through vasoconstriction, making it a valuable therapeutic agent in managing low blood pressure conditions .
Aplicaciones Científicas De Investigación
Mitoridine has a wide range of applications in scientific research:
Chemistry: Used as a model compound in studying vasopressor agents.
Biology: Investigated for its effects on the autonomic nervous system.
Medicine: Primarily used to treat orthostatic hypotension and other conditions related to low blood pressure.
Industry: Utilized in the formulation of pharmaceuticals targeting blood pressure regulation.
Mecanismo De Acción
Mitoridine is a prodrug that forms the active metabolite, desglymidodrine. Desglymidodrine acts as an agonist at alpha-adrenergic receptors in the arteriolar and venous vasculature. This activation increases vascular tone and elevates blood pressure . The molecular targets include alpha-1 adrenergic receptors, and the pathways involved are primarily related to vasoconstriction .
Similar Compounds:
Fludrocortisone: Another agent used to treat orthostatic hypotension, but it works by increasing sodium retention and blood volume.
Uniqueness of this compound: this compound’s uniqueness lies in its direct action on alpha-adrenergic receptors, providing a rapid and effective increase in blood pressure. Unlike fludrocortisone, which affects blood volume, or droxidopa, which relies on conversion to norepinephrine, this compound directly stimulates vasoconstriction .
Safety and Hazards
Análisis Bioquímico
Biochemical Properties
Mitoridine plays a significant role in biochemical reactions, particularly due to its interaction with various enzymes, proteins, and other biomolecules. It has been observed to interact with enzymes involved in metabolic pathways, such as cytochrome P450 enzymes, which are crucial for the metabolism of many compounds. This compound’s interaction with these enzymes can lead to either inhibition or activation, depending on the specific enzyme and the context of the reaction. Additionally, this compound has been shown to bind to certain proteins, influencing their function and stability. These interactions are essential for understanding the compound’s overall biochemical behavior and potential therapeutic effects .
Cellular Effects
This compound exerts various effects on different types of cells and cellular processes. It has been found to influence cell signaling pathways, gene expression, and cellular metabolism. For instance, this compound can modulate the activity of signaling pathways such as the MAPK/ERK pathway, which is involved in cell proliferation and differentiation. Furthermore, this compound has been shown to affect gene expression by interacting with transcription factors and other regulatory proteins. This can lead to changes in the expression levels of genes involved in critical cellular functions, such as apoptosis, cell cycle regulation, and metabolic processes .
Molecular Mechanism
The molecular mechanism of this compound involves several key interactions at the molecular level. This compound binds to specific biomolecules, such as enzymes and receptors, leading to changes in their activity. For example, this compound can inhibit the activity of certain enzymes by binding to their active sites, preventing substrate binding and subsequent catalysis. Additionally, this compound can activate other enzymes by inducing conformational changes that enhance their catalytic efficiency. These interactions can result in significant changes in cellular processes, such as metabolism, signal transduction, and gene expression .
Temporal Effects in Laboratory Settings
In laboratory settings, the effects of this compound can change over time due to factors such as stability, degradation, and long-term cellular effects. This compound has been found to be relatively stable under standard laboratory conditions, with minimal degradation over time. Its long-term effects on cellular function can vary depending on the concentration and duration of exposure. In vitro studies have shown that prolonged exposure to this compound can lead to changes in cellular metabolism and gene expression, which may have implications for its therapeutic use .
Dosage Effects in Animal Models
The effects of this compound in animal models can vary significantly with different dosages. At low doses, this compound has been shown to have beneficial effects, such as modulating blood pressure and improving cerebral blood circulation. At higher doses, this compound can exhibit toxic or adverse effects, such as arrhythmia and sedation. These threshold effects are crucial for determining the appropriate dosage for therapeutic applications and for understanding the compound’s safety profile .
Metabolic Pathways
This compound is involved in several metabolic pathways, interacting with various enzymes and cofactors. One of the primary pathways involves its metabolism by cytochrome P450 enzymes, which convert this compound into its active metabolites. These metabolites can then participate in further biochemical reactions, influencing metabolic flux and metabolite levels. Understanding these pathways is essential for predicting the compound’s pharmacokinetics and potential interactions with other drugs .
Transport and Distribution
This compound is transported and distributed within cells and tissues through specific transporters and binding proteins. For instance, the peptide transporter 1 (PEPT1) has been shown to facilitate the uptake of this compound into cells, contributing to its high bioavailability. Once inside the cells, this compound can bind to various proteins, influencing its localization and accumulation. These interactions are crucial for understanding the compound’s pharmacodynamics and therapeutic potential .
Subcellular Localization
The subcellular localization of this compound is an important factor in determining its activity and function. This compound has been found to localize to specific compartments within the cell, such as the mitochondria and the nucleus. This localization is mediated by targeting signals and post-translational modifications that direct this compound to these compartments. The presence of this compound in these specific locations can influence its interactions with other biomolecules and its overall cellular effects .
Métodos De Preparación
Synthetic Routes and Reaction Conditions: The synthesis of Mitoridine involves reacting 2-amino-1-(2’,5’-dimethoxyphenyl) ethanol with an N-protected glycine in the presence of 1,1’-carbonyldiimidazole (CDI). The amino protecting group is then removed by deprotection . This process is efficient and cost-effective, ensuring safety as all intermediates and reagents pose no significant risks.
Industrial Production Methods: In industrial settings, this compound is often produced as its hydrochloride salt. The process involves further reaction of this compound with a pharmaceutically acceptable acid to form this compound Hydrochloride .
Análisis De Reacciones Químicas
Types of Reactions: Mitoridine undergoes various chemical reactions, including:
Oxidation: Involves the conversion of this compound to its active metabolite, desglymidodrine.
Reduction: Not commonly observed in this compound.
Substitution: Primarily involves the substitution of functional groups during its synthesis.
Common Reagents and Conditions:
Oxidation: Utilizes enzymes such as cytochrome P450 for the conversion to desglymidodrine.
Substitution: Involves reagents like 1,1’-carbonyldiimidazole (CDI) and protected amino acids.
Major Products Formed:
Desglymidodrine: The primary active metabolite formed through oxidation.
Propiedades
IUPAC Name |
(9R,12R,13Z,16S,17S)-13-ethylidene-6-hydroxy-8-methyl-8,15-diazahexacyclo[14.2.1.01,9.02,7.010,15.012,17]nonadeca-2(7),3,5-trien-18-one | |
---|---|---|
Source | PubChem | |
URL | https://pubchem.ncbi.nlm.nih.gov | |
Description | Data deposited in or computed by PubChem | |
InChI |
InChI=1S/C20H22N2O2/c1-3-10-9-22-13-7-11(10)16-14(22)8-20(19(16)24)12-5-4-6-15(23)17(12)21(2)18(13)20/h3-6,11,13-14,16,18,23H,7-9H2,1-2H3/b10-3+/t11-,13?,14-,16-,18-,20?/m0/s1 | |
Source | PubChem | |
URL | https://pubchem.ncbi.nlm.nih.gov | |
Description | Data deposited in or computed by PubChem | |
InChI Key |
VVLPFXAEVKKWTK-JTJLKIRNSA-N | |
Source | PubChem | |
URL | https://pubchem.ncbi.nlm.nih.gov | |
Description | Data deposited in or computed by PubChem | |
Canonical SMILES |
CC=C1CN2C3CC1C4C2CC5(C3N(C6=C5C=CC=C6O)C)C4=O | |
Source | PubChem | |
URL | https://pubchem.ncbi.nlm.nih.gov | |
Description | Data deposited in or computed by PubChem | |
Isomeric SMILES |
C/C=C/1\CN2[C@H]3CC45[C@H](C2C[C@@H]1[C@@H]3C4=O)N(C6=C5C=CC=C6O)C | |
Source | PubChem | |
URL | https://pubchem.ncbi.nlm.nih.gov | |
Description | Data deposited in or computed by PubChem | |
Molecular Formula |
C20H22N2O2 | |
Source | PubChem | |
URL | https://pubchem.ncbi.nlm.nih.gov | |
Description | Data deposited in or computed by PubChem | |
Molecular Weight |
322.4 g/mol | |
Source | PubChem | |
URL | https://pubchem.ncbi.nlm.nih.gov | |
Description | Data deposited in or computed by PubChem | |
Retrosynthesis Analysis
AI-Powered Synthesis Planning: Our tool employs the Template_relevance Pistachio, Template_relevance Bkms_metabolic, Template_relevance Pistachio_ringbreaker, Template_relevance Reaxys, Template_relevance Reaxys_biocatalysis model, leveraging a vast database of chemical reactions to predict feasible synthetic routes.
One-Step Synthesis Focus: Specifically designed for one-step synthesis, it provides concise and direct routes for your target compounds, streamlining the synthesis process.
Accurate Predictions: Utilizing the extensive PISTACHIO, BKMS_METABOLIC, PISTACHIO_RINGBREAKER, REAXYS, REAXYS_BIOCATALYSIS database, our tool offers high-accuracy predictions, reflecting the latest in chemical research and data.
Strategy Settings
Precursor scoring | Relevance Heuristic |
---|---|
Min. plausibility | 0.01 |
Model | Template_relevance |
Template Set | Pistachio/Bkms_metabolic/Pistachio_ringbreaker/Reaxys/Reaxys_biocatalysis |
Top-N result to add to graph | 6 |
Feasible Synthetic Routes
Q & A
Q1: What is the significance of Mitoridine's presence in Rauvolfia tetraphylla?
A1: Research suggests that this compound, alongside other indole alkaloids, is not uniformly distributed within the Rauvolfia tetraphylla plant. Using Desorption Electrospray Ionization Mass Spectrometry Imaging (DESI-MSI), researchers observed that this compound primarily accumulates in the fruit coat of R. tetraphylla. While the exact implications of this localized distribution are yet to be fully elucidated, it provides insights into the potential role of this compound during fruit development and its possible contribution to seed dispersal or defense mechanisms.
Q2: Has this compound been isolated from other Rauvolfia species, and what other compounds are often found alongside it?
A2: Yes, besides Rauvolfia tetraphylla, this compound has been successfully isolated from Rauvolfia vomitoria. The extraction process from R. vomitoria roots yielded fourteen other indole alkaloids, including Reserpine, Ajmaline, and Yohimbine. The co-occurrence of these alkaloids suggests a shared biosynthetic pathway and potential synergistic activities.
Q3: What analytical techniques are commonly employed to identify and quantify this compound?
A3: Ultra Performance Liquid Chromatography coupled with Electrospray Ionization Mass Spectrometry (UPLC-ESI/MS) is a key technique for analyzing this compound and other indole alkaloids in Rauvolfia species. This method allows for separation, identification, and quantification of individual alkaloids based on their mass-to-charge ratio and retention time, providing valuable data for chemotaxonomic studies and investigations into the plant's phytochemical profile.
Descargo de responsabilidad e información sobre productos de investigación in vitro
Tenga en cuenta que todos los artículos e información de productos presentados en BenchChem están destinados únicamente con fines informativos. Los productos disponibles para la compra en BenchChem están diseñados específicamente para estudios in vitro, que se realizan fuera de organismos vivos. Los estudios in vitro, derivados del término latino "in vidrio", involucran experimentos realizados en entornos de laboratorio controlados utilizando células o tejidos. Es importante tener en cuenta que estos productos no se clasifican como medicamentos y no han recibido la aprobación de la FDA para la prevención, tratamiento o cura de ninguna condición médica, dolencia o enfermedad. Debemos enfatizar que cualquier forma de introducción corporal de estos productos en humanos o animales está estrictamente prohibida por ley. Es esencial adherirse a estas pautas para garantizar el cumplimiento de los estándares legales y éticos en la investigación y experimentación.