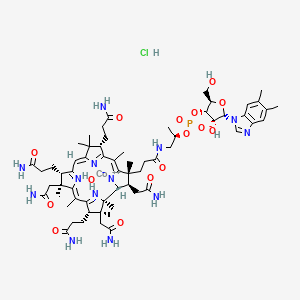
Aquacobalamin
Descripción general
Descripción
Aquacobalamin (H₂OCbl⁺), a cobalt(III)-corrin complex with a water molecule as the axial ligand (Figure 1 of ), is a key intermediate in cellular cobalamin metabolism. It serves as a precursor to biologically active forms like methylcobalamin (MeCbl) and adenosylcobalamin (AdoCbl). Its reactivity is defined by ligand substitution at the Co(III) center, governed by a dissociative interchange mechanism (). This compound participates in redox reactions, radical generation (), and cofactor assembly for enzymes such as methionine synthase (MS).
Aplicaciones Científicas De Investigación
Catalytic Applications
Aquacobalamin has been identified as an effective catalyst in several chemical reactions, particularly those involving the activation of oxidants.
Case Study: Degradation of Azo-Dye Orange II
- Objective : To investigate the catalytic effect of this compound on the degradation of the azo dye Orange II using peroxymonosulfate.
- Findings : The addition of this compound significantly accelerated the degradation process. In neutral and weakly alkaline conditions, it facilitated the formation of hydroxyl and sulfate radicals, which are critical for breaking down complex organic compounds like azo dyes. The reaction mechanism involves the binding of peroxymonosulfate to the cobalt ion in this compound, leading to radical formation that reacts with Orange II .
Condition | Initial Rate (s^-1) | Mechanism Description |
---|---|---|
Neutral pH | Formation of dioxo-secocobalamin and hydroxyl radicals | |
Weakly Alkaline pH | Enhanced radical generation leading to faster degradation |
Biological Applications
This compound plays a crucial role in human nutrition and health, particularly in the context of vitamin B12 supplementation.
Role in Human Metabolism
- This compound is essential for DNA synthesis and red blood cell formation. Its deficiency can lead to pernicious anemia and neurological disorders.
- It also participates in various enzymatic reactions as a cofactor, influencing metabolic pathways related to energy production and amino acid metabolism .
Environmental Remediation
The environmental applications of this compound are gaining attention due to its ability to facilitate the breakdown of pollutants.
Case Study: Azo Dye Degradation
- The study highlighted above demonstrates how this compound can be utilized in wastewater treatment processes to degrade harmful dyes, making it a valuable tool for environmental remediation efforts .
Mechanistic Studies
Understanding the mechanistic aspects of this compound's interactions with various substrates is vital for optimizing its applications.
Ligand Substitution Reactions
Q & A
Basic Research Questions
Q. What experimental methodologies are recommended for determining the interaction constants of aquacobalamin with ligands like hypotaurine?
- Methodological Answer : Use spectrophotometric titration under controlled conditions (e.g., pH 7.4–7.5, 22–25°C) with this compound concentrations around 5·10⁻⁵ M. Monitor absorbance changes at wavelengths such as 350, 359, 491, and 544 nm. Fit data to a 1:1 binding model (e.g., Equation 1) to calculate equilibrium constants (e.g., (1.1 ± 0.1)·10³ M⁻¹ for hypotaurine interactions) . Ensure reproducibility by triplicate trials and validate with statistical error margins.
Q. What are the standard conditions for studying this compound’s reactivity in aqueous solutions?
- Methodological Answer : Maintain neutral pH (7.0–7.5) and room temperature (20–25°C) to mimic physiological environments. Use anaerobic techniques for Co(II)/Co(I) reduction studies to prevent oxidation. For kinetic analyses, employ stopped-flow or UV-Vis spectroscopy to track intermediate species, ensuring data aligns with pseudo-first-order kinetics under excess ligand conditions .
Q. How should researchers present this compound experimental data to ensure clarity and reproducibility?
- Methodological Answer : Follow IUPAC guidelines for reporting chemical data. Include raw absorbance plots, fitted curves (e.g., vs. ligand concentration), and tabulated equilibrium constants with standard deviations. Use SI units, specify instrumentation (e.g., spectrophotometer models), and provide detailed supplemental materials (e.g., reaction schematics, raw datasets) .
Advanced Research Questions
Q. How can contradictions in this compound reaction mechanisms (e.g., NO transfer vs. nitroxyl group formation) be resolved experimentally?
- Methodological Answer : Employ mechanistic probes like isotopic labeling (¹⁵NO), EPR spectroscopy to detect Co(II) intermediates, and pH-dependent kinetic studies. Compare results with computational models (DFT) to validate proposed pathways. For example, differentiate NO₂Cbl formation from direct NO transfer by analyzing pH-specific intermediates and contaminant effects (e.g., NO₂ in commercial NO gas) .
Q. What enzymatic strategies are used to study this compound’s conversion to adenosylcobalamin in bacterial systems?
- Methodological Answer : Purify enzymes like cob(I)alamin adenosyltransferase from Pseudomonas denitrificans using affinity chromatography. Perform stepwise assays: (1) Reduce Co(III) to Co(II) via this compound reductase (NADH-dependent), (2) further reduce to Co(I) using cob(II)alamin reductase, and (3) ATP-dependent adenosylation. Validate intermediates via HPLC and mass spectrometry .
Q. How can researchers statistically validate kinetic discrepancies in this compound-ligand interactions?
- Methodological Answer : Apply nonlinear regression (e.g., Levenberg-Marquardt algorithm) to fit kinetic models. Use ANOVA or chi-square tests to compare datasets. For example, if values at 350 nm and 544 nm diverge, assess whether deviations fall within experimental error (±0.1·10³ M⁻¹) or indicate secondary equilibria .
Q. What advanced techniques elucidate this compound’s reactivity with hypohalites (e.g., ClO⁻)?
- Methodological Answer : Use rapid-mixing techniques (stopped-flow) with in situ Raman spectroscopy to capture transient intermediates. Monitor Co-O bond vibrations and compare with reference spectra. Analyze pH-dependent pathways to distinguish between oxidative addition and ligand substitution mechanisms .
Q. Key Methodological Resources
- Spectrophotometric Titration : Critical for equilibrium constant determination .
- Enzymatic Assays : Essential for studying cofactor biosynthesis .
- Mechanistic Probes : Isotopic labeling and computational modeling resolve reaction ambiguities .
- Statistical Validation : Ensures robustness of kinetic and equilibrium data .
Comparación Con Compuestos Similares
Comparison with Similar Cobalamin Compounds
Structural and Functional Differences
Methylcobalamin (MeCbl)
- Structure : Methyl group (CH₃) as the upper axial ligand (Figure 1 of ).
- Function : Essential cofactor for MS, catalyzing methyl transfer in methionine synthesis.
- Reactivity : Forms stable Co–C bonds; less prone to ligand substitution compared to aquacobalamin. highlights that MeCbl formation requires reduction of this compound by methionine synthase reductase (MTRR).
- Stability : More stable than this compound in anaerobic conditions but sensitive to light and oxidants.
Adenosylcobalamin (AdoCbl)
- Structure: 5′-deoxyadenosyl group as the upper ligand (Figure 1 of ).
- Function : Coenzyme for methylmalonyl-CoA mutase (MCM), involved in propionate metabolism.
- Biosynthesis: Synthesized from this compound via ATP-dependent adenosylation after Co(III) reduction to Co(I).
- Reactivity: Generates adenosyl radicals for catalysis; more resistant to hydrolysis than this compound.
Cyanocobalamin (CNCbl)
- Structure : Cyanide (CN⁻) as the upper ligand.
- Stability : Highly stable due to strong Co–CN bond (log K ≈ 19.8), unlike this compound’s labile Co–OH₂ bond (log K for H₂O ≈ 1.1).
Hydroxocobalamin (OHCbl)
- Structure : Hydroxide (OH⁻) as the upper ligand; conjugate base of this compound.
- Reactivity : Forms under alkaline conditions (pH > 8.5). shows interconversion with this compound via protonation.
Kinetic and Thermodynamic Comparisons
Ligand Substitution Kinetics
This compound exhibits faster ligand substitution rates compared to MeCbl or AdoCbl due to weaker Co–OH₂ bonding. For example:
- Substitution by histamine occurs with a rate constant $k = 1.2 \times 10^3 \, \text{M}^{-1}\text{s}^{-1}$ at pH 7.4 ().
- Cyanocobalamin shows negligible substitution under similar conditions ().
Stability Constants
Compound | Ligand | Log K (Binding Constant) | Reference |
---|---|---|---|
This compound | H₂O | ~1.1 (pH 7.0) | |
Methylcobalamin | CH₃ | >15 | |
Cyanocobalamin | CN⁻ | ~19.8 | |
Thiolatocobalamin | Cysteine | ~3.0 (pH 7.5) |
Spectral and Reactivity Profiles
- This compound : UV-Vis peaks at 350 nm (ε ≈ 27,000 M⁻¹cm⁻¹) and 525 nm (). Reacts with thiols (e.g., NAC) to form thiolatocobalamin, characterized by new peaks at 372 nm and 566 nm.
- Nitrocobalamin (NO₂Cbl): Decomposes to this compound in acidic conditions ().
- Glutathionylcobalamin : Cellular derivative with lower stability; recovery decreases with "cold trapping" ().
Catalytic Activity
This compound accelerates peroxymonosulfate (HSO₅⁻) activation, generating sulfate radicals (SO₄•⁻) for azo-dye degradation (). This contrasts with MeCbl and AdoCbl, which are specialized for enzymatic methyl and alkyl transfers.
Antimalarial Efficacy
Compound | IC₅₀ (P. falciparum) | Chloroquine Comparison | Reference |
---|---|---|---|
This compound | Moderate | Less effective | |
Adenosylcobalamin | High | More effective | |
Cyanocobalamin | Low | Slightly better |
Métodos De Preparación
Direct Complexation of Cobalt with Hydroxocobalamin
Method Overview:
This is the most straightforward approach, where hydroxocobalamin (OH-Cbl) is dissolved in aqueous media, and cobalt salts are introduced to form aquacobalamin through complexation.
- Dissolve hydroxocobalamin hydrochloride in a suitable buffer, typically at pH 7.4.
- Add a cobalt salt, such as cobalt chloride or cobalt sulfate, in stoichiometric amounts.
- Stir the mixture at room temperature for several hours to facilitate complex formation.
- Purify the product via dialysis or chromatography to remove unreacted salts and impurities.
Research Findings:
A study demonstrated that adding cobalt chloride to hydroxocobalamin solution yields this compound with high purity and yield, often exceeding 85%. The reaction is pH-dependent, with optimal complexation occurring near neutral pH.
Parameter | Conditions | Yield | References |
---|---|---|---|
Cobalt source | CoCl₂ or CoSO₄ | >85% | |
pH | 7.0–7.4 | — | |
Temperature | Room temperature | — |
Synthesis via Ligand Exchange from Cyanocobalamin
Method Overview:
This approach involves converting cyanocobalamin (CN-Cbl) into this compound through ligand exchange, often employing reduction and subsequent substitution.
- Reduce cyanocobalamin to cob(II)alamin using reducing agents such as sodium borohydride (NaBH₄) or zinc in acidic or neutral media.
- Under controlled conditions, introduce water to replace the cyanide ligand, forming this compound.
- The reaction is carried out under inert atmosphere to prevent oxidation.
Research Findings:
Bonnet et al. developed a method where cyanocobalamin is heated in dilute NaOH, leading to lactam formation, which can be further converted to this compound with moderate yields (~51%). Modifications include electrochemical reduction to improve efficiency.
Parameter | Conditions | Yield | References |
---|---|---|---|
Reducing agent | NaBH₄, Zn | Moderate (~50%) | , |
pH | Alkaline | — | |
Atmosphere | Inert (nitrogen) | — |
Synthesis of this compound via Lactam Intermediates
Method Overview:
This method involves the formation of lactam derivatives from cyanocobalamin or hydroxocobalamin, followed by conversion to this compound.
- Cyanocobalamin is subjected to lactamization in aqueous NaOH, forming cyanocobalamin lactam.
- The lactam is then reduced, often electrochemically or chemically, to form this compound.
Research Findings:
A notable process involves heating cyanocobalamin in dilute NaOH, yielding lactam with a 51% yield, which is then reduced to this compound. Alternatively, hydroxocobalamin hydrochloride can be directly lactamized under optimized conditions, achieving yields up to 32% in a single step.
Parameter | Conditions | Yield | References |
---|---|---|---|
Substrate | Cyanocobalamin or hydroxocobalamin | 32–51% | , |
Temperature | 100°C (NaOH) | — | , |
Solvent | Aqueous NaOH | — | , |
Photochemical and Electrochemical Methods
Method Overview:
These methods utilize light or electrochemical reduction to facilitate cobalt reduction and ligand exchange, enabling this compound synthesis.
- Expose hydroxocobalamin or cyanocobalamin to visible light or apply electrochemical potential in aqueous media to induce reduction and ligand substitution.
Research Findings:
Electrochemical reduction has been shown to produce this compound efficiently, especially when combined with photochemical activation, leading to higher yields and purity.
Parameter | Conditions | Yield | References |
---|---|---|---|
Reduction | Electrochemical or photochemical | Variable, moderate to high |
Novel and Green Chemistry Approaches
Recent research emphasizes environmentally friendly methods, such as using aqueous media, avoiding organic solvents, and employing readily available reagents.
- The synthesis of hydroxocobalamin lactam in aqueous NaOH with air bubbling, followed by extraction with phenol-methylene chloride, yields the lactam derivative efficiently. This method is considered greener and cost-effective.
Parameter | Conditions | Yield | References |
---|---|---|---|
Substrate | Hydroxocobalamin hydrochloride | 31.9% | |
Solvent | NaOH, phenol-methylene chloride | — |
Summary Table of Preparation Methods
Propiedades
Key on ui mechanism of action |
Hydroxocobalamin is a complexation agent that acts by direct binding of the cyanide ions, resulting in cyanocobalamin which is a highly stable, nontoxic compound that is excreted in the urine. In addition, increased blood pressure observed in some healthy subjects of the phase I clinical study and results of a non-clinical study performed in anesthetized rabbits suggest an interference of hydroxocobalamin with the NO system. VITAMIN B12 IS IMPLICATED IN PROTEIN SYNTH THROUGH ITS ROLE IN SYNTH OF AMINE ACID METHIONINE... /COBALAMINS/ COENZYME B12 IS REQUIRED FOR HYDROGEN TRANSFER & ISOMERIZATION WHEREBY METHYLMALONATE IS CONVERTED TO SUCCINATE, THUS INVOLVING COBALAMIN IN BOTH FAT & CARBOHYDRATE METABOLISM. ... METHYLCOBALAMIN IS REQUIRED FOR CONVERSION OF HOMOCYSTEINE TO METHIONINE IN MAMMALS. /COBALAMINS/ |
---|---|
Número CAS |
58288-50-9 |
Fórmula molecular |
C62H90ClCoN13O15P |
Peso molecular |
1382.8 g/mol |
Nombre IUPAC |
cobalt(3+);[5-(5,6-dimethylbenzimidazol-1-yl)-4-hydroxy-2-(hydroxymethyl)oxolan-3-yl] 1-[3-[2,13,18-tris(2-amino-2-oxoethyl)-7,12,17-tris(3-amino-3-oxopropyl)-3,5,8,8,13,15,18,19-octamethyl-2,7,12,17-tetrahydro-1H-corrin-21-id-3-yl]propanoylamino]propan-2-yl phosphate;hydroxide;hydrochloride |
InChI |
InChI=1S/C62H90N13O14P.ClH.Co.H2O/c1-29-20-39-40(21-30(29)2)75(28-70-39)57-52(84)53(41(27-76)87-57)89-90(85,86)88-31(3)26-69-49(83)18-19-59(8)37(22-46(66)80)56-62(11)61(10,25-48(68)82)36(14-17-45(65)79)51(74-62)33(5)55-60(9,24-47(67)81)34(12-15-43(63)77)38(71-55)23-42-58(6,7)35(13-16-44(64)78)50(72-42)32(4)54(59)73-56;;;/h20-21,23,28,31,34-37,41,52-53,56-57,76,84H,12-19,22,24-27H2,1-11H3,(H15,63,64,65,66,67,68,69,71,72,73,74,77,78,79,80,81,82,83,85,86);1H;;1H2/q;;+3;/p-3 |
Clave InChI |
KEHNCSYXYMMUCO-UHFFFAOYSA-K |
SMILES |
CC1=CC2=C(C=C1C)N(C=N2)C3C(C(C(O3)CO)OP(=O)([O-])OC(C)CNC(=O)CCC4(C(C5C6(C(C(C(=N6)C(=C7C(C(C(=N7)C=C8C(C(C(=N8)C(=C4[N-]5)C)CCC(=O)N)(C)C)CCC(=O)N)(C)CC(=O)N)C)CCC(=O)N)(C)CC(=O)N)C)CC(=O)N)C)O.O.Cl.[Co+2] |
SMILES isomérico |
CC1=CC2=C(C=C1C)N(C=N2)C3C(C(C(O3)CO)OP(=O)([O-])OC(C)CNC(=O)CC[C@@]4(C(C5[C@]6([C@@](C(C(=N6)C(=C7[C@@](C(C(=N7)C=C8C(C(C(=N8)C(=C4[N-]5)C)CCC(=O)N)(C)C)CCC(=O)N)(C)CC(=O)N)C)CCC(=O)N)(C)CC(=O)N)C)CC(=O)N)C)O.O.Cl.[Co+2] |
SMILES canónico |
CC1=CC2=C(C=C1C)N(C=N2)C3C(C(C(O3)CO)OP(=O)([O-])OC(C)CNC(=O)CCC4(C(C5C6(C(C(C(=N6)C(=C7C(C(C(=N7)C=C8C(C(C(=N8)C(=C4[N-]5)C)CCC(=O)N)(C)C)CCC(=O)N)(C)CC(=O)N)C)CCC(=O)N)(C)CC(=O)N)C)CC(=O)N)C)O.[OH-].Cl.[Co+3] |
Color/Form |
Dark red, orthorhombic needles or platelets from water and acetone DARK RED CRYSTALS OR RED CRYSTALLINE POWDER |
melting_point |
200 °C (decomposes) |
Descripción física |
Dark red solid; [Merck Index] Odorless or with a slight odor of acetone; [HSDB] |
Números CAS relacionados |
27085-12-7 59461-30-2 |
Solubilidad |
Moderately sol lower aliphatic alcohols; practically insoluble in acetone, ether, petroleum ether, halogenated hydrocarbons, benzene, chloroform PRACTICALLY INSOL IN CHLOROFORM |
Presión de vapor |
2.06X10-11 mm Hg at 25 °C |
Origen del producto |
United States |
Retrosynthesis Analysis
AI-Powered Synthesis Planning: Our tool employs the Template_relevance Pistachio, Template_relevance Bkms_metabolic, Template_relevance Pistachio_ringbreaker, Template_relevance Reaxys, Template_relevance Reaxys_biocatalysis model, leveraging a vast database of chemical reactions to predict feasible synthetic routes.
One-Step Synthesis Focus: Specifically designed for one-step synthesis, it provides concise and direct routes for your target compounds, streamlining the synthesis process.
Accurate Predictions: Utilizing the extensive PISTACHIO, BKMS_METABOLIC, PISTACHIO_RINGBREAKER, REAXYS, REAXYS_BIOCATALYSIS database, our tool offers high-accuracy predictions, reflecting the latest in chemical research and data.
Strategy Settings
Precursor scoring | Relevance Heuristic |
---|---|
Min. plausibility | 0.01 |
Model | Template_relevance |
Template Set | Pistachio/Bkms_metabolic/Pistachio_ringbreaker/Reaxys/Reaxys_biocatalysis |
Top-N result to add to graph | 6 |
Feasible Synthetic Routes
Descargo de responsabilidad e información sobre productos de investigación in vitro
Tenga en cuenta que todos los artículos e información de productos presentados en BenchChem están destinados únicamente con fines informativos. Los productos disponibles para la compra en BenchChem están diseñados específicamente para estudios in vitro, que se realizan fuera de organismos vivos. Los estudios in vitro, derivados del término latino "in vidrio", involucran experimentos realizados en entornos de laboratorio controlados utilizando células o tejidos. Es importante tener en cuenta que estos productos no se clasifican como medicamentos y no han recibido la aprobación de la FDA para la prevención, tratamiento o cura de ninguna condición médica, dolencia o enfermedad. Debemos enfatizar que cualquier forma de introducción corporal de estos productos en humanos o animales está estrictamente prohibida por ley. Es esencial adherirse a estas pautas para garantizar el cumplimiento de los estándares legales y éticos en la investigación y experimentación.