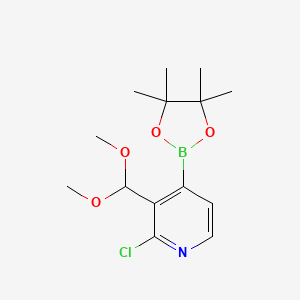
2-Cloro-3-(dimetoxi-metil)-4-(4,4,5,5-tetrametil-1,3,2-dioxaborolan-2-il)piridina
Descripción general
Descripción
2-Chloro-3-(dimethoxymethyl)-4-(4,4,5,5-tetramethyl-1,3,2-dioxaborolan-2-yl)pyridine is a complex organic compound featuring a pyridine ring substituted with a chloro group, a dimethoxymethyl group, and a boronate ester
Aplicaciones Científicas De Investigación
Chemistry
In organic synthesis, 2-Chloro-3-(dimethoxymethyl)-4-(4,4,5,5-tetramethyl-1,3,2-dioxaborolan-2-yl)pyridine is used as a building block for the synthesis of more complex molecules. Its boronate ester group makes it a valuable reagent in cross-coupling reactions, facilitating the formation of diverse carbon-carbon bonds.
Biology and Medicine
This compound can be used in the development of pharmaceuticals, particularly in the synthesis of drug candidates that require the pyridine scaffold
Industry
In the industrial sector, this compound can be used in the production of agrochemicals, dyes, and materials science applications. Its versatility in chemical reactions makes it a valuable intermediate in the synthesis of complex organic molecules.
Métodos De Preparación
Synthetic Routes and Reaction Conditions
The synthesis of 2-Chloro-3-(dimethoxymethyl)-4-(4,4,5,5-tetramethyl-1,3,2-dioxaborolan-2-yl)pyridine typically involves multiple steps:
Formation of the Pyridine Ring: The pyridine ring can be synthesized through various methods, including the Hantzsch pyridine synthesis, which involves the condensation of β-ketoesters with ammonia and aldehydes.
Introduction of the Chloro Group: Chlorination of the pyridine ring can be achieved using reagents such as thionyl chloride or phosphorus pentachloride.
Boronate Ester Formation: The final step involves the formation of the boronate ester, which can be achieved by reacting the pyridine derivative with bis(pinacolato)diboron under palladium-catalyzed conditions.
Industrial Production Methods
Industrial production of this compound would likely follow similar synthetic routes but on a larger scale, with optimizations for yield and purity. This may involve continuous flow chemistry techniques and the use of automated reactors to ensure consistent production.
Análisis De Reacciones Químicas
Types of Reactions
Substitution Reactions: The chloro group in the compound can undergo nucleophilic substitution reactions, where it is replaced by other nucleophiles such as amines or thiols.
Oxidation and Reduction: The dimethoxymethyl group can be oxidized to form aldehydes or carboxylic acids, while reduction can yield alcohols.
Cross-Coupling Reactions: The boronate ester group is particularly useful in Suzuki-Miyaura cross-coupling reactions, allowing the formation of carbon-carbon bonds with various aryl or vinyl halides.
Common Reagents and Conditions
Nucleophilic Substitution: Reagents like sodium azide or potassium thiolate in polar aprotic solvents.
Oxidation: Reagents such as potassium permanganate or chromium trioxide.
Reduction: Reagents like lithium aluminum hydride or sodium borohydride.
Cross-Coupling: Palladium catalysts, typically in the presence of bases like potassium carbonate and solvents such as toluene or ethanol.
Major Products
Substitution: Formation of substituted pyridine derivatives.
Oxidation: Aldehydes or carboxylic acids.
Reduction: Alcohols.
Cross-Coupling: Biaryl or vinyl-aryl compounds.
Mecanismo De Acción
The mechanism of action of 2-Chloro-3-(dimethoxymethyl)-4-(4,4,5,5-tetramethyl-1,3,2-dioxaborolan-2-yl)pyridine depends on the specific application and the chemical transformations it undergoes. In cross-coupling reactions, the boronate ester group participates in the formation of a palladium complex, which facilitates the transfer of the aryl or vinyl group to the coupling partner.
Comparación Con Compuestos Similares
Similar Compounds
2-Chloro-3-(methoxymethyl)-4-(4,4,5,5-tetramethyl-1,3,2-dioxaborolan-2-yl)pyridine: Similar structure but with a methoxymethyl group instead of a dimethoxymethyl group.
2-Chloro-3-(dimethoxymethyl)-4-(phenyl)-pyridine: Similar structure but with a phenyl group instead of the boronate ester.
Uniqueness
The unique combination of the chloro, dimethoxymethyl, and boronate ester groups in 2-Chloro-3-(dimethoxymethyl)-4-(4,4,5,5-tetramethyl-1,3,2-dioxaborolan-2-yl)pyridine provides a versatile platform for various chemical transformations. This makes it particularly valuable in synthetic organic chemistry for the construction of complex molecules.
This detailed overview should provide a comprehensive understanding of 2-Chloro-3-(dimethoxymethyl)-4-(4,4,5,5-tetramethyl-1,3,2-dioxaborolan-2-yl)pyridine, its preparation, reactions, applications, and comparison with similar compounds
Actividad Biológica
2-Chloro-3-(dimethoxymethyl)-4-(4,4,5,5-tetramethyl-1,3,2-dioxaborolan-2-yl)pyridine is a synthetic compound with significant potential in biological applications. This article delves into its biological activity, including mechanisms of action, therapeutic potential, and relevant research findings.
Chemical Structure and Properties
- Molecular Formula : C14H21BClNO4
- Molecular Weight : 313.6 g/mol
- CAS Number : 1315341-84-4
The compound features a pyridine ring substituted with a chloro group and a boron-containing moiety. Its unique structure contributes to its biological properties.
The biological activity of this compound is primarily attributed to its ability to interact with specific biological targets. The dioxaborolane group is known for its role in forming covalent bonds with biomolecules, which can lead to modulation of enzyme activity and influence cellular signaling pathways.
Biological Activity Overview
Research has indicated that 2-Chloro-3-(dimethoxymethyl)-4-(4,4,5,5-tetramethyl-1,3,2-dioxaborolan-2-yl)pyridine exhibits several biological activities:
- Anticancer Activity : Preliminary studies suggest that this compound may inhibit the growth of certain cancer cell lines by inducing apoptosis and disrupting cell cycle progression.
- Protein Degradation : It is being explored as a potential protein degrader in targeted protein degradation (TPD) strategies. The boron atom in its structure is crucial for the formation of stable complexes with target proteins.
- Enzyme Inhibition : The compound has shown promise in inhibiting specific enzymes involved in metabolic pathways.
Table 1: Summary of Biological Activities
Case Study: Anticancer Effects
A study published in Journal of Medicinal Chemistry evaluated the anticancer properties of this compound on various cancer cell lines. Results indicated a significant reduction in cell viability at concentrations above 10 µM, with mechanisms involving apoptosis activation and cell cycle arrest observed through flow cytometry analysis.
Case Study: Protein Targeting
In another investigation focused on protein degradation, researchers employed 2-Chloro-3-(dimethoxymethyl)-4-(4,4,5,5-tetramethyl-1,3,2-dioxaborolan-2-yl)pyridine as part of a bifunctional degrader system. The study demonstrated effective degradation of target proteins in cellular models, highlighting its potential utility in therapeutic applications targeting oncogenic proteins.
Propiedades
IUPAC Name |
2-chloro-3-(dimethoxymethyl)-4-(4,4,5,5-tetramethyl-1,3,2-dioxaborolan-2-yl)pyridine | |
---|---|---|
Source | PubChem | |
URL | https://pubchem.ncbi.nlm.nih.gov | |
Description | Data deposited in or computed by PubChem | |
InChI |
InChI=1S/C14H21BClNO4/c1-13(2)14(3,4)21-15(20-13)9-7-8-17-11(16)10(9)12(18-5)19-6/h7-8,12H,1-6H3 | |
Source | PubChem | |
URL | https://pubchem.ncbi.nlm.nih.gov | |
Description | Data deposited in or computed by PubChem | |
InChI Key |
QYVUCDAMZFZENI-UHFFFAOYSA-N | |
Source | PubChem | |
URL | https://pubchem.ncbi.nlm.nih.gov | |
Description | Data deposited in or computed by PubChem | |
Canonical SMILES |
B1(OC(C(O1)(C)C)(C)C)C2=C(C(=NC=C2)Cl)C(OC)OC | |
Source | PubChem | |
URL | https://pubchem.ncbi.nlm.nih.gov | |
Description | Data deposited in or computed by PubChem | |
Molecular Formula |
C14H21BClNO4 | |
Source | PubChem | |
URL | https://pubchem.ncbi.nlm.nih.gov | |
Description | Data deposited in or computed by PubChem | |
Molecular Weight |
313.59 g/mol | |
Source | PubChem | |
URL | https://pubchem.ncbi.nlm.nih.gov | |
Description | Data deposited in or computed by PubChem | |
Retrosynthesis Analysis
AI-Powered Synthesis Planning: Our tool employs the Template_relevance Pistachio, Template_relevance Bkms_metabolic, Template_relevance Pistachio_ringbreaker, Template_relevance Reaxys, Template_relevance Reaxys_biocatalysis model, leveraging a vast database of chemical reactions to predict feasible synthetic routes.
One-Step Synthesis Focus: Specifically designed for one-step synthesis, it provides concise and direct routes for your target compounds, streamlining the synthesis process.
Accurate Predictions: Utilizing the extensive PISTACHIO, BKMS_METABOLIC, PISTACHIO_RINGBREAKER, REAXYS, REAXYS_BIOCATALYSIS database, our tool offers high-accuracy predictions, reflecting the latest in chemical research and data.
Strategy Settings
Precursor scoring | Relevance Heuristic |
---|---|
Min. plausibility | 0.01 |
Model | Template_relevance |
Template Set | Pistachio/Bkms_metabolic/Pistachio_ringbreaker/Reaxys/Reaxys_biocatalysis |
Top-N result to add to graph | 6 |
Feasible Synthetic Routes
Descargo de responsabilidad e información sobre productos de investigación in vitro
Tenga en cuenta que todos los artículos e información de productos presentados en BenchChem están destinados únicamente con fines informativos. Los productos disponibles para la compra en BenchChem están diseñados específicamente para estudios in vitro, que se realizan fuera de organismos vivos. Los estudios in vitro, derivados del término latino "in vidrio", involucran experimentos realizados en entornos de laboratorio controlados utilizando células o tejidos. Es importante tener en cuenta que estos productos no se clasifican como medicamentos y no han recibido la aprobación de la FDA para la prevención, tratamiento o cura de ninguna condición médica, dolencia o enfermedad. Debemos enfatizar que cualquier forma de introducción corporal de estos productos en humanos o animales está estrictamente prohibida por ley. Es esencial adherirse a estas pautas para garantizar el cumplimiento de los estándares legales y éticos en la investigación y experimentación.