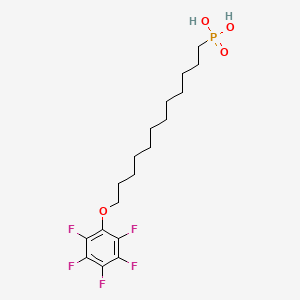
12-(2,3,4,5,6-Pentafluorophenoxy)dodecylphosphonic acid
Descripción general
Descripción
“12-(2,3,4,5,6-Pentafluorophenoxy)dodecylphosphonic acid” is a compound used in scientific research. It possesses unique properties, making it valuable for various applications such as surface modification and catalysis. The compound is also known as P-[12-(2,3,4,5,6-Pentafluorophenoxy)dodecyl]phosphonic acid .
Molecular Structure Analysis
The empirical formula of the compound is C18H26F5O4P . The molecular weight is 432.36 . The SMILES string representation of the molecule is OP(O)(=O)CCCCCCCCCCCCOc1c(F)c(F)c(F)c(F)c1F .
Physical And Chemical Properties Analysis
The compound is in powder form . It has a melting point of 80-85 °C .
Aplicaciones Científicas De Investigación
Overview of Phosphonic Acids in Research Phosphonic acids, characterized by a phosphorus atom bonded to three oxygen atoms and one carbon atom, are utilized across a wide array of applications due to their structural analogy with the phosphate moiety or their coordination or supramolecular properties. These compounds find applications in bioactive properties (drug, pro-drug), bone targeting, design of supramolecular or hybrid materials, surface functionalization, analytical purposes, medical imaging, or as phosphoantigens. Their applications span chemistry, biology, and physics, highlighting the synthesis of phosphonic acids as critical for numerous research projects. The methods for synthesizing phosphonic acids vary, including dealkylation of dialkyl phosphonates under acidic conditions or the McKenna procedure, demonstrating their versatility and importance in research (Sevrain, Berchel, Couthon, & Jaffrès, 2017).
Degradation of Polymers by Phosphonic and Phosphoric Acids Phosphonic and phosphoric acids have been identified as effective agents for the degradation of polymer wastes, including polyurethanes, polycarbonates, and polyamides. Research has shown that alkyl esters of H-phosphonic and phosphoric acids can significantly degrade polymers, leading to products with fire retardant properties. This opens new avenues for recycling and repurposing materials, showcasing the environmental and functional applications of phosphonic acids in material science (Mitova, Grancharov, Molero, Borreguero, Troev, & Rodríguez, 2013).
Phosphonate-Based Antiscalants in Water Treatment In the realm of water treatment, phosphonates serve as crucial antiscalants, preventing the precipitation of salts on reverse osmosis membranes. This application underscores the role of phosphonic acids in maintaining the efficiency and longevity of water purification systems. Technical investigations have revealed the presence of undeclared phosphorous contaminants in phosphonate-based antiscalants, emphasizing the importance of thorough characterization and regulation in environmental and public health contexts (Armbruster, Müller, & Happel, 2019).
Phosphonopeptides in Medicine and Agriculture Phosphonopeptides, where a phosphonic acid group replaces a carboxylic acid group or mimics peptide bonds, have emerged as potent inhibitors of key enzymes related to various pathological states. Their physiological activities offer potential applications in medicine and agriculture, demonstrating the biochemical and therapeutic significance of phosphonic acid derivatives (Kafarski, 2020).
Environmental Implications of Polyfluoroalkyl Chemicals The environmental fate of polyfluoroalkyl chemicals, which often contain phosphonic acid groups, has been a subject of intensive study due to their persistence and toxicological profiles. Research into microbial degradation and the development of novel intermediates provides insight into the challenges and strategies for mitigating the environmental impact of these substances (Liu & Mejia Avendaño, 2013).
Safety And Hazards
The compound is classified as Eye Irritant 2, Skin Irritant 2, and STOT SE 3 . It may cause respiratory irritation, skin irritation, and serious eye irritation . Safety measures include avoiding breathing dust/fume/gas/mist/vapors/spray, washing thoroughly after handling, using only outdoors or in a well-ventilated area, wearing protective gloves/protective clothing/eye protection/face protection, and if on skin: washing with plenty of soap and water .
Propiedades
IUPAC Name |
12-(2,3,4,5,6-pentafluorophenoxy)dodecylphosphonic acid | |
---|---|---|
Source | PubChem | |
URL | https://pubchem.ncbi.nlm.nih.gov | |
Description | Data deposited in or computed by PubChem | |
InChI |
InChI=1S/C18H26F5O4P/c19-13-14(20)16(22)18(17(23)15(13)21)27-11-9-7-5-3-1-2-4-6-8-10-12-28(24,25)26/h1-12H2,(H2,24,25,26) | |
Source | PubChem | |
URL | https://pubchem.ncbi.nlm.nih.gov | |
Description | Data deposited in or computed by PubChem | |
InChI Key |
ULXYEINUTVZCLX-UHFFFAOYSA-N | |
Source | PubChem | |
URL | https://pubchem.ncbi.nlm.nih.gov | |
Description | Data deposited in or computed by PubChem | |
Canonical SMILES |
C(CCCCCCP(=O)(O)O)CCCCCOC1=C(C(=C(C(=C1F)F)F)F)F | |
Source | PubChem | |
URL | https://pubchem.ncbi.nlm.nih.gov | |
Description | Data deposited in or computed by PubChem | |
Molecular Formula |
C18H26F5O4P | |
Source | PubChem | |
URL | https://pubchem.ncbi.nlm.nih.gov | |
Description | Data deposited in or computed by PubChem | |
Molecular Weight |
432.4 g/mol | |
Source | PubChem | |
URL | https://pubchem.ncbi.nlm.nih.gov | |
Description | Data deposited in or computed by PubChem | |
Product Name |
12-Pentafluorophenoxydodecylphosphonic acid |
Retrosynthesis Analysis
AI-Powered Synthesis Planning: Our tool employs the Template_relevance Pistachio, Template_relevance Bkms_metabolic, Template_relevance Pistachio_ringbreaker, Template_relevance Reaxys, Template_relevance Reaxys_biocatalysis model, leveraging a vast database of chemical reactions to predict feasible synthetic routes.
One-Step Synthesis Focus: Specifically designed for one-step synthesis, it provides concise and direct routes for your target compounds, streamlining the synthesis process.
Accurate Predictions: Utilizing the extensive PISTACHIO, BKMS_METABOLIC, PISTACHIO_RINGBREAKER, REAXYS, REAXYS_BIOCATALYSIS database, our tool offers high-accuracy predictions, reflecting the latest in chemical research and data.
Strategy Settings
Precursor scoring | Relevance Heuristic |
---|---|
Min. plausibility | 0.01 |
Model | Template_relevance |
Template Set | Pistachio/Bkms_metabolic/Pistachio_ringbreaker/Reaxys/Reaxys_biocatalysis |
Top-N result to add to graph | 6 |
Feasible Synthetic Routes
Descargo de responsabilidad e información sobre productos de investigación in vitro
Tenga en cuenta que todos los artículos e información de productos presentados en BenchChem están destinados únicamente con fines informativos. Los productos disponibles para la compra en BenchChem están diseñados específicamente para estudios in vitro, que se realizan fuera de organismos vivos. Los estudios in vitro, derivados del término latino "in vidrio", involucran experimentos realizados en entornos de laboratorio controlados utilizando células o tejidos. Es importante tener en cuenta que estos productos no se clasifican como medicamentos y no han recibido la aprobación de la FDA para la prevención, tratamiento o cura de ninguna condición médica, dolencia o enfermedad. Debemos enfatizar que cualquier forma de introducción corporal de estos productos en humanos o animales está estrictamente prohibida por ley. Es esencial adherirse a estas pautas para garantizar el cumplimiento de los estándares legales y éticos en la investigación y experimentación.