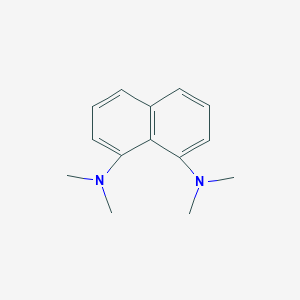
1,8-Bis(dimethylamino)naphthalene
Descripción general
Descripción
1,8-Bis(dimethylamino)naphthalene, also referred to as proton sponge, is an organic compound with the formula C10H6(NMe2)2 (Me = methyl). It is classified as a peri-naphthalene, i.e., a 1,8-disubstituted derivative of naphthalene . It exhibits exceptional basicity due to its unusual structure . It is often referred to by the trade name Proton Sponge, a trademark of Sigma-Aldrich .
Molecular Structure Analysis
This compound is a diamine in which the two dimethylamino groups are attached on the same side (peri position) of a naphthalene ring . The 1,8-bis(dimethylamino)naphthalene cation has mirror symmetry, with the mirror plane passing along the C(9)-C(10) bond. The two N atoms are moved slightly out of the naphthalene plane .
Chemical Reactions Analysis
1,8-Bis(dimethylamino)naphthalene is a very strong base with weak nucleophilic character due to steric effects . It has been used in organic synthesis as a highly selective non-nucleophilic base . It also exhibits a very high affinity for boron and is capable of displacing hydride from borane to form a boronium–borohydride ion pair .
Physical And Chemical Properties Analysis
1,8-Bis(dimethylamino)naphthalene is a white crystalline powder . It has a density of 1.1±0.1 g/cm3, a boiling point of 304.4±22.0 °C at 760 mmHg, and a flash point of 128.3±10.2 °C .
Aplicaciones Científicas De Investigación
Synthesis of C-substituted t-BuNH-8,9-R,R′-nido-7,8,9-C3B8H9 Tricarbollide Compounds
1,8-Bis(dimethylamino)naphthalene is used in the synthesis of C-substituted t-BuNH-8,9-R,R′-nido-7,8,9-C3B8H9 (R,R′ = H,H; MeH; Me,Me; Ph,H and Ph,Ph) tricarbollide compounds . These compounds have potential applications in the field of organometallic chemistry.
Preparation of Saturated Fluoroalkyl (Hydrido) Complexes of Iridium
This compound has also been used in the preparation of saturated fluoroalkyl (hydrido) complexes of Iridium . These complexes are of interest in the field of inorganic chemistry, particularly in the study of transition metal complexes.
Mecanismo De Acción
1,8-Bis(dimethylamino)naphthalene, also known as N1,N1,N8,N8-tetramethylnaphthalene-1,8-diamine or Proton Sponge, is an organic compound with a unique structure that exhibits exceptional basicity .
Target of Action
The primary target of 1,8-Bis(dimethylamino)naphthalene is the proton (H+). It is often referred to as a Proton Sponge due to its ability to absorb protons . This compound is a diamine in which the two dimethylamino groups are attached on the same side (peri position) of a naphthalene ring .
Mode of Action
The compound interacts with its target (protons) by absorbing them slowly. The high basicity of this compound is attributed to the relief of strain upon protonation and/or the strong interaction between the nitrogen lone pairs . It is sterically hindered, making it a weak nucleophile .
Safety and Hazards
Propiedades
IUPAC Name |
1-N,1-N,8-N,8-N-tetramethylnaphthalene-1,8-diamine | |
---|---|---|
Source | PubChem | |
URL | https://pubchem.ncbi.nlm.nih.gov | |
Description | Data deposited in or computed by PubChem | |
InChI |
InChI=1S/C14H18N2/c1-15(2)12-9-5-7-11-8-6-10-13(14(11)12)16(3)4/h5-10H,1-4H3 | |
Source | PubChem | |
URL | https://pubchem.ncbi.nlm.nih.gov | |
Description | Data deposited in or computed by PubChem | |
InChI Key |
GJFNRSDCSTVPCJ-UHFFFAOYSA-N | |
Source | PubChem | |
URL | https://pubchem.ncbi.nlm.nih.gov | |
Description | Data deposited in or computed by PubChem | |
Canonical SMILES |
CN(C)C1=CC=CC2=C1C(=CC=C2)N(C)C | |
Source | PubChem | |
URL | https://pubchem.ncbi.nlm.nih.gov | |
Description | Data deposited in or computed by PubChem | |
Molecular Formula |
C14H18N2 | |
Source | PubChem | |
URL | https://pubchem.ncbi.nlm.nih.gov | |
Description | Data deposited in or computed by PubChem | |
DSSTOX Substance ID |
DTXSID10174807 | |
Record name | 1,8-Bis(dimethylamino)naphthalene | |
Source | EPA DSSTox | |
URL | https://comptox.epa.gov/dashboard/DTXSID10174807 | |
Description | DSSTox provides a high quality public chemistry resource for supporting improved predictive toxicology. | |
Molecular Weight |
214.31 g/mol | |
Source | PubChem | |
URL | https://pubchem.ncbi.nlm.nih.gov | |
Description | Data deposited in or computed by PubChem | |
Physical Description |
Pale cream crystalline powder; [Alfa Aesar MSDS] | |
Record name | 1,8-Bis(dimethylamino)naphthalene | |
Source | Haz-Map, Information on Hazardous Chemicals and Occupational Diseases | |
URL | https://haz-map.com/Agents/19299 | |
Description | Haz-Map® is an occupational health database designed for health and safety professionals and for consumers seeking information about the adverse effects of workplace exposures to chemical and biological agents. | |
Explanation | Copyright (c) 2022 Haz-Map(R). All rights reserved. Unless otherwise indicated, all materials from Haz-Map are copyrighted by Haz-Map(R). No part of these materials, either text or image may be used for any purpose other than for personal use. Therefore, reproduction, modification, storage in a retrieval system or retransmission, in any form or by any means, electronic, mechanical or otherwise, for reasons other than personal use, is strictly prohibited without prior written permission. | |
Solubility |
31.2 [ug/mL] (The mean of the results at pH 7.4) | |
Record name | SID26727059 | |
Source | Burnham Center for Chemical Genomics | |
URL | https://pubchem.ncbi.nlm.nih.gov/bioassay/1996#section=Data-Table | |
Description | Aqueous solubility in buffer at pH 7.4 | |
Product Name |
1,8-Bis(dimethylamino)naphthalene | |
CAS RN |
20734-58-1 | |
Record name | Proton sponge | |
Source | CAS Common Chemistry | |
URL | https://commonchemistry.cas.org/detail?cas_rn=20734-58-1 | |
Description | CAS Common Chemistry is an open community resource for accessing chemical information. Nearly 500,000 chemical substances from CAS REGISTRY cover areas of community interest, including common and frequently regulated chemicals, and those relevant to high school and undergraduate chemistry classes. This chemical information, curated by our expert scientists, is provided in alignment with our mission as a division of the American Chemical Society. | |
Explanation | The data from CAS Common Chemistry is provided under a CC-BY-NC 4.0 license, unless otherwise stated. | |
Record name | 1,8-Bis(dimethylamino)naphthalene | |
Source | ChemIDplus | |
URL | https://pubchem.ncbi.nlm.nih.gov/substance/?source=chemidplus&sourceid=0020734581 | |
Description | ChemIDplus is a free, web search system that provides access to the structure and nomenclature authority files used for the identification of chemical substances cited in National Library of Medicine (NLM) databases, including the TOXNET system. | |
Record name | 1,8-Bis(dimethylamino)naphthalene | |
Source | EPA DSSTox | |
URL | https://comptox.epa.gov/dashboard/DTXSID10174807 | |
Description | DSSTox provides a high quality public chemistry resource for supporting improved predictive toxicology. | |
Record name | N,N,N',N'-tetramethylnaphthalene-1,8-diamine | |
Source | European Chemicals Agency (ECHA) | |
URL | https://echa.europa.eu/substance-information/-/substanceinfo/100.039.986 | |
Description | The European Chemicals Agency (ECHA) is an agency of the European Union which is the driving force among regulatory authorities in implementing the EU's groundbreaking chemicals legislation for the benefit of human health and the environment as well as for innovation and competitiveness. | |
Explanation | Use of the information, documents and data from the ECHA website is subject to the terms and conditions of this Legal Notice, and subject to other binding limitations provided for under applicable law, the information, documents and data made available on the ECHA website may be reproduced, distributed and/or used, totally or in part, for non-commercial purposes provided that ECHA is acknowledged as the source: "Source: European Chemicals Agency, http://echa.europa.eu/". Such acknowledgement must be included in each copy of the material. ECHA permits and encourages organisations and individuals to create links to the ECHA website under the following cumulative conditions: Links can only be made to webpages that provide a link to the Legal Notice page. | |
Record name | 1,8-BIS(DIMETHYLAMINO)NAPHTHALENE | |
Source | FDA Global Substance Registration System (GSRS) | |
URL | https://gsrs.ncats.nih.gov/ginas/app/beta/substances/6S79D2P9H8 | |
Description | The FDA Global Substance Registration System (GSRS) enables the efficient and accurate exchange of information on what substances are in regulated products. Instead of relying on names, which vary across regulatory domains, countries, and regions, the GSRS knowledge base makes it possible for substances to be defined by standardized, scientific descriptions. | |
Explanation | Unless otherwise noted, the contents of the FDA website (www.fda.gov), both text and graphics, are not copyrighted. They are in the public domain and may be republished, reprinted and otherwise used freely by anyone without the need to obtain permission from FDA. Credit to the U.S. Food and Drug Administration as the source is appreciated but not required. | |
Retrosynthesis Analysis
AI-Powered Synthesis Planning: Our tool employs the Template_relevance Pistachio, Template_relevance Bkms_metabolic, Template_relevance Pistachio_ringbreaker, Template_relevance Reaxys, Template_relevance Reaxys_biocatalysis model, leveraging a vast database of chemical reactions to predict feasible synthetic routes.
One-Step Synthesis Focus: Specifically designed for one-step synthesis, it provides concise and direct routes for your target compounds, streamlining the synthesis process.
Accurate Predictions: Utilizing the extensive PISTACHIO, BKMS_METABOLIC, PISTACHIO_RINGBREAKER, REAXYS, REAXYS_BIOCATALYSIS database, our tool offers high-accuracy predictions, reflecting the latest in chemical research and data.
Strategy Settings
Precursor scoring | Relevance Heuristic |
---|---|
Min. plausibility | 0.01 |
Model | Template_relevance |
Template Set | Pistachio/Bkms_metabolic/Pistachio_ringbreaker/Reaxys/Reaxys_biocatalysis |
Top-N result to add to graph | 6 |
Feasible Synthetic Routes
Descargo de responsabilidad e información sobre productos de investigación in vitro
Tenga en cuenta que todos los artículos e información de productos presentados en BenchChem están destinados únicamente con fines informativos. Los productos disponibles para la compra en BenchChem están diseñados específicamente para estudios in vitro, que se realizan fuera de organismos vivos. Los estudios in vitro, derivados del término latino "in vidrio", involucran experimentos realizados en entornos de laboratorio controlados utilizando células o tejidos. Es importante tener en cuenta que estos productos no se clasifican como medicamentos y no han recibido la aprobación de la FDA para la prevención, tratamiento o cura de ninguna condición médica, dolencia o enfermedad. Debemos enfatizar que cualquier forma de introducción corporal de estos productos en humanos o animales está estrictamente prohibida por ley. Es esencial adherirse a estas pautas para garantizar el cumplimiento de los estándares legales y éticos en la investigación y experimentación.