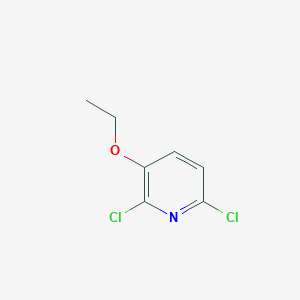
2,6-Dichloro-3-ethoxypyridine
Descripción general
Descripción
2,6-Dichloro-3-ethoxypyridine is a heterocyclic compound belonging to the pyridine family. It is characterized by the presence of two chlorine atoms and an ethoxy group attached to a pyridine ring. The molecular formula of this compound is C7H7Cl2NO, and it has a molecular weight of 192.04 g/mol . This compound is a pale yellow crystalline solid and is used in various fields, including medical, environmental, and industrial research.
Métodos De Preparación
Synthetic Routes and Reaction Conditions: 2,6-Dichloro-3-ethoxypyridine can be synthesized through the reaction of bromoethane with 2,6-dichloropyridin-3-ol . The reaction typically involves the use of a base such as potassium carbonate in an organic solvent like dimethylformamide (DMF) under reflux conditions. The reaction proceeds through nucleophilic substitution, where the ethoxy group replaces the hydroxyl group on the pyridine ring.
Industrial Production Methods: Industrial production of this compound follows similar synthetic routes but on a larger scale. The reaction conditions are optimized to ensure high yield and purity. The product is then purified through recrystallization or distillation to meet industrial standards.
Análisis De Reacciones Químicas
Types of Reactions: 2,6-Dichloro-3-ethoxypyridine undergoes various chemical reactions, including:
Nucleophilic Substitution: The chlorine atoms on the pyridine ring can be replaced by nucleophiles such as amines, thiols, or alkoxides.
Oxidation: The ethoxy group can be oxidized to form corresponding aldehydes or carboxylic acids.
Reduction: The compound can be reduced to form this compound derivatives with different functional groups.
Common Reagents and Conditions:
Nucleophilic Substitution: Reagents like sodium methoxide, potassium tert-butoxide, or primary amines in solvents like ethanol or DMF.
Oxidation: Oxidizing agents such as potassium permanganate or chromium trioxide in acidic or basic conditions.
Reduction: Reducing agents like lithium aluminum hydride or sodium borohydride in anhydrous solvents.
Major Products:
Nucleophilic Substitution: Formation of substituted pyridines with various functional groups.
Oxidation: Formation of aldehydes or carboxylic acids.
Reduction: Formation of reduced pyridine derivatives.
Aplicaciones Científicas De Investigación
2,6-Dichloro-3-ethoxypyridine has a wide range of applications in scientific research:
Chemistry: Used as an intermediate in the synthesis of complex organic molecules and pharmaceuticals.
Biology: Investigated for its potential biological activities, including antimicrobial and anticancer properties.
Medicine: Explored for its potential use in drug development and as a building block for active pharmaceutical ingredients.
Industry: Utilized in the production of agrochemicals, dyes, and other industrial chemicals
Mecanismo De Acción
The mechanism of action of 2,6-Dichloro-3-ethoxypyridine depends on its specific application. In biological systems, it may interact with molecular targets such as enzymes or receptors, leading to inhibition or activation of specific pathways. For example, it may inhibit bacterial growth by interfering with essential enzymes or disrupt cancer cell proliferation by targeting specific signaling pathways .
Comparación Con Compuestos Similares
2,6-Dichloropyridine: Lacks the ethoxy group, making it less versatile in certain chemical reactions.
3-Ethoxypyridine: Lacks the chlorine atoms, resulting in different reactivity and applications.
2,6-Dichloro-4-ethoxypyridine: Similar structure but with the ethoxy group at a different position, leading to different chemical properties.
Uniqueness: 2,6-Dichloro-3-ethoxypyridine is unique due to the presence of both chlorine atoms and the ethoxy group on the pyridine ring. This combination of functional groups provides a balance of reactivity and stability, making it a valuable intermediate in various chemical syntheses and applications .
Propiedades
IUPAC Name |
2,6-dichloro-3-ethoxypyridine | |
---|---|---|
Source | PubChem | |
URL | https://pubchem.ncbi.nlm.nih.gov | |
Description | Data deposited in or computed by PubChem | |
InChI |
InChI=1S/C7H7Cl2NO/c1-2-11-5-3-4-6(8)10-7(5)9/h3-4H,2H2,1H3 | |
Source | PubChem | |
URL | https://pubchem.ncbi.nlm.nih.gov | |
Description | Data deposited in or computed by PubChem | |
InChI Key |
WGULPAIELXHVIG-UHFFFAOYSA-N | |
Source | PubChem | |
URL | https://pubchem.ncbi.nlm.nih.gov | |
Description | Data deposited in or computed by PubChem | |
Canonical SMILES |
CCOC1=C(N=C(C=C1)Cl)Cl | |
Source | PubChem | |
URL | https://pubchem.ncbi.nlm.nih.gov | |
Description | Data deposited in or computed by PubChem | |
Molecular Formula |
C7H7Cl2NO | |
Source | PubChem | |
URL | https://pubchem.ncbi.nlm.nih.gov | |
Description | Data deposited in or computed by PubChem | |
Molecular Weight |
192.04 g/mol | |
Source | PubChem | |
URL | https://pubchem.ncbi.nlm.nih.gov | |
Description | Data deposited in or computed by PubChem | |
Retrosynthesis Analysis
AI-Powered Synthesis Planning: Our tool employs the Template_relevance Pistachio, Template_relevance Bkms_metabolic, Template_relevance Pistachio_ringbreaker, Template_relevance Reaxys, Template_relevance Reaxys_biocatalysis model, leveraging a vast database of chemical reactions to predict feasible synthetic routes.
One-Step Synthesis Focus: Specifically designed for one-step synthesis, it provides concise and direct routes for your target compounds, streamlining the synthesis process.
Accurate Predictions: Utilizing the extensive PISTACHIO, BKMS_METABOLIC, PISTACHIO_RINGBREAKER, REAXYS, REAXYS_BIOCATALYSIS database, our tool offers high-accuracy predictions, reflecting the latest in chemical research and data.
Strategy Settings
Precursor scoring | Relevance Heuristic |
---|---|
Min. plausibility | 0.01 |
Model | Template_relevance |
Template Set | Pistachio/Bkms_metabolic/Pistachio_ringbreaker/Reaxys/Reaxys_biocatalysis |
Top-N result to add to graph | 6 |
Feasible Synthetic Routes
Descargo de responsabilidad e información sobre productos de investigación in vitro
Tenga en cuenta que todos los artículos e información de productos presentados en BenchChem están destinados únicamente con fines informativos. Los productos disponibles para la compra en BenchChem están diseñados específicamente para estudios in vitro, que se realizan fuera de organismos vivos. Los estudios in vitro, derivados del término latino "in vidrio", involucran experimentos realizados en entornos de laboratorio controlados utilizando células o tejidos. Es importante tener en cuenta que estos productos no se clasifican como medicamentos y no han recibido la aprobación de la FDA para la prevención, tratamiento o cura de ninguna condición médica, dolencia o enfermedad. Debemos enfatizar que cualquier forma de introducción corporal de estos productos en humanos o animales está estrictamente prohibida por ley. Es esencial adherirse a estas pautas para garantizar el cumplimiento de los estándares legales y éticos en la investigación y experimentación.