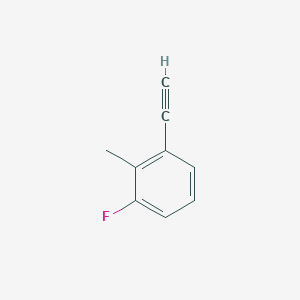
1-Ethynyl-3-fluoro-2-methylbenzene
Descripción general
Descripción
1-Ethynyl-3-fluoro-2-methylbenzene (CAS: 176896-72-3) is a halogenated aromatic compound characterized by a benzene ring substituted with an ethynyl group (-C≡CH) at position 1, a fluorine atom at position 3, and a methyl group at position 2. Its structural uniqueness lies in the combination of electron-withdrawing (fluoro) and electron-donating (methyl) substituents, alongside the highly reactive ethynyl moiety. However, its discontinued status has hindered extensive experimental characterization.
Métodos De Preparación
Synthetic Routes and Reaction Conditions: 1-Ethynyl-3-fluoro-2-methylbenzene can be synthesized through a multi-step process involving electrophilic aromatic substitution reactions. One common method involves the following steps:
Friedel-Crafts Acylation:
Reduction: Conversion of the acyl group to an alkane using a reducing agent like zinc amalgam in hydrochloric acid (Clemmensen reduction).
Halogenation: Introduction of a fluorine atom via halogenation using fluorine gas or a fluorinating agent.
Alkyne Formation: Introduction of the ethynyl group through a Sonogashira coupling reaction, which involves the coupling of an aryl halide with an alkyne in the presence of a palladium catalyst and a copper co-catalyst
Industrial Production Methods: Industrial production of this compound typically involves large-scale application of the aforementioned synthetic routes, with optimization for yield and purity. Continuous flow reactors and automated synthesis platforms are often employed to enhance efficiency and scalability.
Análisis De Reacciones Químicas
Types of Reactions: 1-Ethynyl-3-fluoro-2-methylbenzene undergoes various types of chemical reactions, including:
Electrophilic Aromatic Substitution: Reactions such as nitration, sulfonation, and halogenation.
Nucleophilic Substitution: Reactions involving the replacement of the fluorine atom with nucleophiles.
Addition Reactions: Reactions involving the addition of reagents to the ethynyl group, such as hydrogenation and halogenation.
Common Reagents and Conditions:
Nitration: Concentrated nitric acid and sulfuric acid.
Sulfonation: Fuming sulfuric acid.
Halogenation: Halogen gas (e.g., fluorine, chlorine) or halogenating agents.
Hydrogenation: Hydrogen gas in the presence of a palladium or platinum catalyst.
Major Products Formed:
Nitration: Formation of nitro derivatives.
Sulfonation: Formation of sulfonic acid derivatives.
Hydrogenation: Formation of ethyl-substituted derivatives.
Aplicaciones Científicas De Investigación
Common Synthesis Methods
Method | Description |
---|---|
Electrophilic Aromatic Substitution | Substitution of hydrogen atoms on the benzene ring with electrophiles. |
Friedel-Crafts Acylation | Acylation of benzene derivatives using acyl chlorides in the presence of Lewis acids. |
Organic Synthesis
1-Ethynyl-3-fluoro-2-methylbenzene serves as a crucial building block in organic synthesis, enabling the formation of more complex molecules. Its reactivity allows for various transformations, including:
- Oxidation: Producing carbonyl compounds or carboxylic acids.
- Reduction: Converting the vinyl group to an ethyl group.
- Substitution Reactions: Introducing new substituents onto the aromatic ring.
Medicinal Chemistry
The compound has shown promise in medicinal chemistry, particularly in studies related to enzyme inhibition and receptor binding assays. Its structural features enhance its binding affinity to biological targets, making it a candidate for drug development.
Case Study: Anticancer Properties
Research indicates that derivatives of this compound can inhibit specific cancer-related enzymes. For instance, compounds similar to this have demonstrated inhibitory effects on Sirtuin 2 (SIRT2), which is implicated in cancer progression.
Material Science
In material science, this compound is utilized in the production of polymers and resins with specific electronic properties. Its unique structure allows for modifications that enhance material characteristics, such as thermal stability and mechanical strength.
The biological activity of this compound is attributed to its ability to undergo electrophilic aromatic substitution reactions. The presence of the vinyl group increases reactivity, while the fluorine atom enhances lipophilicity, improving interactions with biological targets.
Key Mechanisms
- Electrophilic Aromatic Substitution: Facilitates interactions with electrophiles.
- Hydrophobic Interactions: The fluorine atom enhances binding affinity.
- Enzyme Interactions: Useful in metabolic pathway studies.
Mecanismo De Acción
The mechanism of action of 1-ethynyl-3-fluoro-2-methylbenzene involves its interaction with various molecular targets and pathways. The ethynyl group can participate in cycloaddition reactions, forming triazoles in the presence of azides under copper-catalyzed conditions. The fluorine atom can influence the compound’s reactivity and binding affinity through inductive and resonance effects, affecting its interaction with biological targets .
Comparación Con Compuestos Similares
Comparison with Structurally Similar Compounds
To contextualize the properties and reactivity of 1-ethynyl-3-fluoro-2-methylbenzene, a comparative analysis with analogous halogenated benzenes is provided below.
Structural Analogues from Discontinued Products
The following table compares this compound with other discontinued halogenated benzenes, focusing on substituent patterns and functional groups:
Key Observations :
- Reactivity: The ethynyl group in the target compound contrasts with the bromo substituent in 1-(2-bromophenyl)-3-oxocyclobutane-1-carboxylic acid. Ethynyl groups are typically reactive in Sonogashira couplings, whereas bromo substituents participate in Ullmann or Suzuki-Miyaura reactions.
Actividad Biológica
1-Ethynyl-3-fluoro-2-methylbenzene, also known as a fluorinated aromatic compound, has garnered attention in various fields of research due to its unique structural properties and potential biological activities. This article explores its biological activity, including mechanisms of action, therapeutic potential, and relevant case studies.
Chemical Structure and Properties
This compound features a phenyl ring substituted with an ethynyl group at one position, a fluorine atom at another, and a methyl group. Its chemical structure can be represented as follows:
This compound exhibits lipophilicity due to the presence of the ethynyl and methyl groups, which may enhance its ability to penetrate biological membranes.
The biological activity of this compound is primarily attributed to its interaction with specific biological targets. The mechanisms include:
- Enzyme Inhibition : The compound may act as an inhibitor for various enzymes, modulating biochemical pathways. Studies have shown that fluorinated compounds often exhibit increased binding affinity due to the electronegative nature of fluorine, which can stabilize interactions with active sites on enzymes .
- Receptor Modulation : It may interact with receptor proteins, influencing signaling pathways. This modulation can lead to altered cellular responses that are beneficial in therapeutic contexts .
Anticancer Activity
Research indicates that compounds similar to this compound have shown promising anticancer properties. For instance, the presence of ethynyl and fluorine groups can enhance the compound's ability to inhibit tumor growth by interfering with cancer cell proliferation pathways .
Case Study:
A study evaluating various fluorinated compounds demonstrated that those with ethynyl substitutions exhibited significant cytotoxic effects on cancer cell lines, including MCF-7 (breast cancer) and HeLa (cervical cancer) cells. The IC50 values ranged from 10 µM to 50 µM depending on structural modifications .
Neuroprotective Effects
Emerging research suggests potential neuroprotective effects of this compound. The compound may influence neuroinflammatory pathways and protect against neuronal cell death in models of neurodegenerative diseases.
Case Study:
In vitro studies indicated that derivatives of this compound could reduce oxidative stress markers in neuronal cell cultures exposed to neurotoxins. This suggests a protective mechanism that warrants further investigation in vivo .
Comparative Analysis of Similar Compounds
To understand the unique properties of this compound, it is useful to compare it with structurally related compounds:
Compound Name | Structure Features | Biological Activity |
---|---|---|
This compound | Ethynyl and fluoro substituents | Anticancer, neuroprotective |
1-Ethynyl-3-chloro-2-methylbenzene | Ethynyl and chloro substituents | Moderate anticancer activity |
4-Fluorophenol | Fluorine on phenolic ring | Antimicrobial properties |
This table highlights how variations in substituent groups can significantly impact biological activity.
Q & A
Basic Questions
Q. What are the optimal synthetic routes for preparing 1-ethynyl-3-fluoro-2-methylbenzene, and how do reaction conditions influence yield and purity?
Methodological Answer: The synthesis of this compound typically involves Sonogashira coupling or dehydrohalogenation of precursor halides. For example, a modified Sonogashira protocol (e.g., using Pd(PPh₃)₄, CuI, and a base like Et₃N) can couple a fluorinated aryl halide with trimethylsilylacetylene, followed by desilylation . Reaction conditions (temperature, solvent polarity, and catalyst loading) critically affect yield: higher temperatures (80–100°C) improve kinetics but may promote side reactions like alkyne oligomerization. Purity is optimized via column chromatography (silica gel, hexane/ethyl acetate gradient) and verified by GC-MS or NMR (e.g., absence of residual Pd in H NMR) .
Q. What spectroscopic techniques are most effective for characterizing this compound, and how are data interpreted?
Methodological Answer:
- NMR : NMR identifies fluorine environment (δ ≈ -110 to -120 ppm for meta-F), while H NMR resolves methyl (δ 2.3–2.5 ppm) and ethynyl protons (δ 3.1–3.3 ppm, if terminal). NMR confirms sp-hybridized carbons (δ 70–85 ppm for C≡C) .
- IR : Alkyne C≡C stretch (~2100 cm⁻¹) and aromatic C-F (1220–1150 cm⁻¹) are diagnostic .
- HRMS : Exact mass analysis (e.g., ESI-TOF) confirms molecular formula (C₉H₇F) with error <2 ppm .
Q. How does the compound’s stability vary under different storage conditions, and what precautions are necessary for long-term use?
Methodological Answer: this compound is prone to oxidation and moisture-induced degradation. Stability tests under inert atmospheres (Ar/N₂) show <5% decomposition after 6 months at -20°C, whereas ambient air leads to 20% degradation (HPLC analysis). Store in amber vials with molecular sieves; monitor via periodic NMR to detect alkyne oxidation (e.g., ketone formation) .
Advanced Research Questions
Q. How can computational modeling (DFT, MD) predict the reactivity of this compound in cross-coupling reactions?
Methodological Answer: Density Functional Theory (DFT) calculates frontier molecular orbitals (HOMO/LUMO) to predict regioselectivity. For example, Fukui indices identify electrophilic sites (C-1 ethynyl position) for Pd-catalyzed couplings. Molecular Dynamics (MD) simulations in solvent models (e.g., THF) reveal steric effects from the methyl and fluorine groups, which hinder catalyst access. Validate predictions with kinetic studies (e.g., monitoring coupling rates via in situ IR) .
Q. What strategies resolve contradictions in experimental vs. theoretical data (e.g., unexpected byproducts in fluorinated alkyne reactions)?
Methodological Answer: Contradictions often arise from unaccounted intermediates or solvent effects. For example, if GC-MS detects a dichlorinated byproduct not predicted by DFT:
Use LC-MS/MS to isolate and characterize the impurity.
Re-run simulations with explicit solvent molecules (e.g., water traces in THF) to identify hidden pathways.
Design control experiments (e.g., anhydrous conditions) to test hypotheses. Cross-disciplinary collaboration with computational chemists is critical .
Q. How can the compound’s electronic and steric properties be exploited in designing novel metal-organic frameworks (MOFs) or catalysts?
Methodological Answer: The ethynyl group acts as a π-donor ligand for transition metals (e.g., Au, Cu), while fluorine enhances MOF thermal stability. Methodological steps:
Synthesize Au(I)-alkyne complexes and characterize via X-ray crystallography.
Test catalytic activity in cycloadditions (e.g., Huisgen reaction) under varying pressures.
Use BET surface area analysis and PXRD to assess MOF porosity and stability .
Q. What advanced techniques (e.g., in situ spectroscopy, microfluidics) are suitable for studying the compound’s reactivity in real-time?
Methodological Answer:
- In situ FTIR : Monitor alkyne consumption during reactions (e.g., C≡C peak decay at 2100 cm⁻¹).
- Microfluidic reactors : Enable precise control of residence time and temperature, revealing transient intermediates via UV-Vis or Raman.
- Cryo-TEM : Capture nanoscale aggregates formed during catalytic cycles. Pair with kinetic modeling (e.g., Arrhenius plots) to derive activation parameters .
Propiedades
IUPAC Name |
1-ethynyl-3-fluoro-2-methylbenzene | |
---|---|---|
Source | PubChem | |
URL | https://pubchem.ncbi.nlm.nih.gov | |
Description | Data deposited in or computed by PubChem | |
InChI |
InChI=1S/C9H7F/c1-3-8-5-4-6-9(10)7(8)2/h1,4-6H,2H3 | |
Source | PubChem | |
URL | https://pubchem.ncbi.nlm.nih.gov | |
Description | Data deposited in or computed by PubChem | |
InChI Key |
PDXPQTPSACFEJE-UHFFFAOYSA-N | |
Source | PubChem | |
URL | https://pubchem.ncbi.nlm.nih.gov | |
Description | Data deposited in or computed by PubChem | |
Canonical SMILES |
CC1=C(C=CC=C1F)C#C | |
Source | PubChem | |
URL | https://pubchem.ncbi.nlm.nih.gov | |
Description | Data deposited in or computed by PubChem | |
Molecular Formula |
C9H7F | |
Source | PubChem | |
URL | https://pubchem.ncbi.nlm.nih.gov | |
Description | Data deposited in or computed by PubChem | |
Molecular Weight |
134.15 g/mol | |
Source | PubChem | |
URL | https://pubchem.ncbi.nlm.nih.gov | |
Description | Data deposited in or computed by PubChem | |
Retrosynthesis Analysis
AI-Powered Synthesis Planning: Our tool employs the Template_relevance Pistachio, Template_relevance Bkms_metabolic, Template_relevance Pistachio_ringbreaker, Template_relevance Reaxys, Template_relevance Reaxys_biocatalysis model, leveraging a vast database of chemical reactions to predict feasible synthetic routes.
One-Step Synthesis Focus: Specifically designed for one-step synthesis, it provides concise and direct routes for your target compounds, streamlining the synthesis process.
Accurate Predictions: Utilizing the extensive PISTACHIO, BKMS_METABOLIC, PISTACHIO_RINGBREAKER, REAXYS, REAXYS_BIOCATALYSIS database, our tool offers high-accuracy predictions, reflecting the latest in chemical research and data.
Strategy Settings
Precursor scoring | Relevance Heuristic |
---|---|
Min. plausibility | 0.01 |
Model | Template_relevance |
Template Set | Pistachio/Bkms_metabolic/Pistachio_ringbreaker/Reaxys/Reaxys_biocatalysis |
Top-N result to add to graph | 6 |
Feasible Synthetic Routes
Descargo de responsabilidad e información sobre productos de investigación in vitro
Tenga en cuenta que todos los artículos e información de productos presentados en BenchChem están destinados únicamente con fines informativos. Los productos disponibles para la compra en BenchChem están diseñados específicamente para estudios in vitro, que se realizan fuera de organismos vivos. Los estudios in vitro, derivados del término latino "in vidrio", involucran experimentos realizados en entornos de laboratorio controlados utilizando células o tejidos. Es importante tener en cuenta que estos productos no se clasifican como medicamentos y no han recibido la aprobación de la FDA para la prevención, tratamiento o cura de ninguna condición médica, dolencia o enfermedad. Debemos enfatizar que cualquier forma de introducción corporal de estos productos en humanos o animales está estrictamente prohibida por ley. Es esencial adherirse a estas pautas para garantizar el cumplimiento de los estándares legales y éticos en la investigación y experimentación.