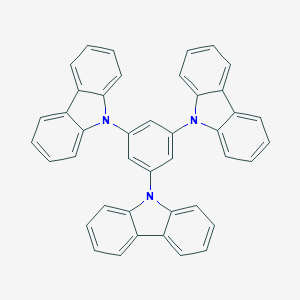
1,3,5-Tri(9H-carbazol-9-yl)benzene
Descripción general
Descripción
1,3,5-Tri(9H-carbazol-9-yl)benzene is a compound with three carbazole units attached to a benzene ring. This structure makes it electron-rich and an excellent hole-transporting layer material. It is primarily used as a phosphorescent host material for light-emitting diodes .
Aplicaciones Científicas De Investigación
1,3,5-Tri(9H-carbazol-9-yl)benzene has a wide range of scientific research applications:
Biology: The compound’s photophysical properties make it useful in biological imaging and as a fluorescent probe.
Medicine: Research is ongoing into its potential use in drug delivery systems and as a component in therapeutic agents.
Mecanismo De Acción
Target of Action
The primary target of 1,3,5-Tri(9H-carbazol-9-yl)benzene, also known as tCP, is the electron transport layer in organic light-emitting diodes (OLEDs) . The compound’s electron-rich nature makes it an excellent hole-transporting layer (HTL) material .
Mode of Action
tCP interacts with its target by facilitating the transport of holes (positive charges) from the anode to the emissive layer in OLEDs . This is due to the electron-rich nature of the carbazole units attached to the benzene ring .
Pharmacokinetics
The term pharmacokinetics is typically used to describe the ADME (Absorption, Distribution, Metabolism, and Excretion) properties of a drug within a biological system Instead, we can discuss the compound’s physical properties. tCP is a solid at room temperature and is soluble in tetrahydrofuran . It has a melting point of 325-330°C .
Result of Action
The result of tCP’s action is the efficient transport of holes to the emissive layer in OLEDs, leading to effective light emission . This makes tCP an excellent material for use in high-efficiency OLEDs .
Action Environment
The performance of tCP, like other OLED materials, can be influenced by environmental factors such as temperature and humidity. For optimal performance and stability, tCP should be stored in a cool, dry environment . Furthermore, the efficiency and lifespan of OLEDs can be significantly affected by water and oxygen, so devices incorporating tCP are usually encapsulated to prevent exposure to these elements .
Análisis Bioquímico
Biochemical Properties
1,3,5-Tri(9H-carbazol-9-yl)benzene is an excellent hole-transporting layer (HTL) material . It interacts with other biomolecules in the context of light-emitting diodes, where it plays a crucial role in the transport of electrons .
Cellular Effects
The effects of this compound on cellular processes are primarily observed in the context of its application in light-emitting diodes
Molecular Mechanism
The molecular mechanism of this compound is related to its role as a hole-transporting layer (HTL) material . It facilitates the transport of electrons, contributing to the functioning of light-emitting diodes .
Temporal Effects in Laboratory Settings
It is known to be a stable compound used in the production of light-emitting diodes .
Metabolic Pathways
Its primary known function is as a hole-transporting layer (HTL) material in the context of light-emitting diodes .
Transport and Distribution
This compound is known to play a role in the transport of electrons in light-emitting diodes
Subcellular Localization
Its known applications are primarily in the field of electronics, specifically in light-emitting diodes .
Métodos De Preparación
Synthetic Routes and Reaction Conditions
1,3,5-Tri(9H-carbazol-9-yl)benzene can be synthesized through a carbazolylation reaction. This typically involves reacting a benzene ring with carbazole-containing compounds and electrophilic reagents to form the desired product . The reaction conditions often include the use of solvents like tetrahydrofuran and temperatures around 325-330°C .
Industrial Production Methods
Industrial production of this compound involves similar synthetic routes but on a larger scale. The compound is purified by sublimation to achieve high purity levels, often greater than 98% as determined by high-performance liquid chromatography (HPLC) .
Análisis De Reacciones Químicas
Types of Reactions
1,3,5-Tri(9H-carbazol-9-yl)benzene undergoes various types of chemical reactions, including:
Oxidation: The compound can be oxidized under specific conditions to form different oxidation products.
Reduction: It can also undergo reduction reactions, although these are less common.
Common Reagents and Conditions
Common reagents used in these reactions include oxidizing agents like potassium permanganate for oxidation and reducing agents like lithium aluminum hydride for reduction. Substitution reactions often involve electrophiles such as halogens or nitro groups .
Major Products
The major products formed from these reactions depend on the specific conditions and reagents used. For example, oxidation may yield carbazole derivatives with additional oxygen-containing functional groups, while substitution reactions can introduce various substituents onto the benzene ring .
Comparación Con Compuestos Similares
Similar Compounds
1,3-Di(9H-carbazol-9-yl)benzene: Similar in structure but with only two carbazole units, making it less efficient in hole transport.
1,2,3,5-Tetrakis(carbazol-9-yl)-4,6-dicyanobenzene: A more complex structure with additional functional groups, used as an organophotocatalyst.
Uniqueness
1,3,5-Tri(9H-carbazol-9-yl)benzene stands out due to its balance of three carbazole units, providing optimal electron-rich properties for hole transport while maintaining structural simplicity. This makes it particularly effective in applications like OLEDs and other electronic devices .
Actividad Biológica
1,3,5-Tri(9H-carbazol-9-yl)benzene (tCP) is a compound notable for its unique structure comprising three carbazole units connected to a benzene ring. This configuration imparts significant electron-rich properties, making it particularly effective in various applications, especially in organic electronics. This article explores the biological activity of tCP, focusing on its biochemical properties, cellular effects, and potential applications in medicine and biology.
- Chemical Formula : C₄₂H₂₇N₃
- Molecular Weight : 573.68 g/mol
- Melting Point : 330 °C
- Purity : >98% (by HPLC) .
The compound is characterized by its high stability and solubility in organic solvents such as tetrahydrofuran (THF), which is critical for its application in organic light-emitting diodes (OLEDs).
Target and Mode of Action
The primary biological target of tCP is the electron transport layer in OLEDs, where it functions as an excellent hole-transporting layer (HTL) material. The mechanism involves facilitating the transport of holes from the anode to the emissive layer, which is crucial for efficient light emission .
tCP exhibits several biochemical properties that are relevant to its function:
- Excellent Hole Transporting Properties : Its structure allows for efficient charge transport, essential for the performance of OLEDs.
- Stability : The compound is stable under laboratory conditions and does not decompose easily, which is advantageous for long-term applications .
Biological Imaging and Fluorescent Probes
Research indicates that tCP's photophysical properties make it suitable for use in biological imaging and as a fluorescent probe . Its ability to emit light at specific wavelengths when excited opens avenues for applications in cellular imaging techniques .
Drug Delivery Systems
Ongoing studies are investigating the potential of tCP as a component in drug delivery systems . Its structural properties may facilitate the encapsulation and controlled release of therapeutic agents .
Comparative Analysis with Similar Compounds
To understand tCP's unique position in biological applications, it is helpful to compare it with structurally similar compounds:
Compound Name | Structure Description | Key Features |
---|---|---|
1,3-Di(9H-carbazol-9-yl)benzene | Two carbazole units | Less efficient hole transport compared to tCP |
1,2,3,5-Tetrakis(carbazol-9-yl)4,6-dicyanobenzene | More complex with additional functional groups | Used as an organophotocatalyst |
tCP stands out due to its optimal balance of three carbazole units that enhance its electron-rich characteristics while maintaining simplicity in structure .
Case Studies and Research Findings
Recent studies have focused on the synthesis and characterization of tCP and its derivatives. For example:
- Photophysical Characterization : Research has demonstrated that tCP has absorption maxima at 292 nm and fluorescence emission at 358 nm when dissolved in THF. These properties are crucial for its application in OLED technology .
- Stability Tests : Long-term stability tests have shown that tCP retains its efficacy as a hole-transporting material even under varying environmental conditions, making it suitable for commercial applications .
- Potential Toxicity Assessments : Preliminary toxicological evaluations suggest that while tCP is effective in electronic applications, further studies are necessary to fully understand its safety profile when applied in biological contexts .
Propiedades
IUPAC Name |
9-[3,5-di(carbazol-9-yl)phenyl]carbazole | |
---|---|---|
Source | PubChem | |
URL | https://pubchem.ncbi.nlm.nih.gov | |
Description | Data deposited in or computed by PubChem | |
InChI |
InChI=1S/C42H27N3/c1-7-19-37-31(13-1)32-14-2-8-20-38(32)43(37)28-25-29(44-39-21-9-3-15-33(39)34-16-4-10-22-40(34)44)27-30(26-28)45-41-23-11-5-17-35(41)36-18-6-12-24-42(36)45/h1-27H | |
Source | PubChem | |
URL | https://pubchem.ncbi.nlm.nih.gov | |
Description | Data deposited in or computed by PubChem | |
InChI Key |
DVNOWTJCOPZGQA-UHFFFAOYSA-N | |
Source | PubChem | |
URL | https://pubchem.ncbi.nlm.nih.gov | |
Description | Data deposited in or computed by PubChem | |
Canonical SMILES |
C1=CC=C2C(=C1)C3=CC=CC=C3N2C4=CC(=CC(=C4)N5C6=CC=CC=C6C7=CC=CC=C75)N8C9=CC=CC=C9C1=CC=CC=C18 | |
Source | PubChem | |
URL | https://pubchem.ncbi.nlm.nih.gov | |
Description | Data deposited in or computed by PubChem | |
Molecular Formula |
C42H27N3 | |
Source | PubChem | |
URL | https://pubchem.ncbi.nlm.nih.gov | |
Description | Data deposited in or computed by PubChem | |
DSSTOX Substance ID |
DTXSID00623490 | |
Record name | 9,9',9''-(Benzene-1,3,5-triyl)tri(9H-carbazole) | |
Source | EPA DSSTox | |
URL | https://comptox.epa.gov/dashboard/DTXSID00623490 | |
Description | DSSTox provides a high quality public chemistry resource for supporting improved predictive toxicology. | |
Molecular Weight |
573.7 g/mol | |
Source | PubChem | |
URL | https://pubchem.ncbi.nlm.nih.gov | |
Description | Data deposited in or computed by PubChem | |
CAS No. |
148044-07-9 | |
Record name | 9,9',9''-(Benzene-1,3,5-triyl)tri(9H-carbazole) | |
Source | EPA DSSTox | |
URL | https://comptox.epa.gov/dashboard/DTXSID00623490 | |
Description | DSSTox provides a high quality public chemistry resource for supporting improved predictive toxicology. | |
Retrosynthesis Analysis
AI-Powered Synthesis Planning: Our tool employs the Template_relevance Pistachio, Template_relevance Bkms_metabolic, Template_relevance Pistachio_ringbreaker, Template_relevance Reaxys, Template_relevance Reaxys_biocatalysis model, leveraging a vast database of chemical reactions to predict feasible synthetic routes.
One-Step Synthesis Focus: Specifically designed for one-step synthesis, it provides concise and direct routes for your target compounds, streamlining the synthesis process.
Accurate Predictions: Utilizing the extensive PISTACHIO, BKMS_METABOLIC, PISTACHIO_RINGBREAKER, REAXYS, REAXYS_BIOCATALYSIS database, our tool offers high-accuracy predictions, reflecting the latest in chemical research and data.
Strategy Settings
Precursor scoring | Relevance Heuristic |
---|---|
Min. plausibility | 0.01 |
Model | Template_relevance |
Template Set | Pistachio/Bkms_metabolic/Pistachio_ringbreaker/Reaxys/Reaxys_biocatalysis |
Top-N result to add to graph | 6 |
Feasible Synthetic Routes
Q1: Why is 1,3,5-Tri(9H-carbazol-9-yl)benzene considered a promising material for blue phosphorescent organic light-emitting diodes (OLEDs)?
A: this compound (TCzP) possesses several properties that make it desirable as a host material in blue phosphorescent OLEDs []:
Q2: How does the structure of this compound contribute to its catalytic activity?
A: The structure of this compound makes it a suitable building block for porous conjugated microporous polymers (CMPs) [, ]. These CMPs exhibit:
Q3: How is this compound used to create effective catalyst supports?
A: this compound serves as a monomer in the synthesis of porous polycarbazole, a material capable of encapsulating and stabilizing metal nanoparticles []. This encapsulation offers several benefits:
Descargo de responsabilidad e información sobre productos de investigación in vitro
Tenga en cuenta que todos los artículos e información de productos presentados en BenchChem están destinados únicamente con fines informativos. Los productos disponibles para la compra en BenchChem están diseñados específicamente para estudios in vitro, que se realizan fuera de organismos vivos. Los estudios in vitro, derivados del término latino "in vidrio", involucran experimentos realizados en entornos de laboratorio controlados utilizando células o tejidos. Es importante tener en cuenta que estos productos no se clasifican como medicamentos y no han recibido la aprobación de la FDA para la prevención, tratamiento o cura de ninguna condición médica, dolencia o enfermedad. Debemos enfatizar que cualquier forma de introducción corporal de estos productos en humanos o animales está estrictamente prohibida por ley. Es esencial adherirse a estas pautas para garantizar el cumplimiento de los estándares legales y éticos en la investigación y experimentación.