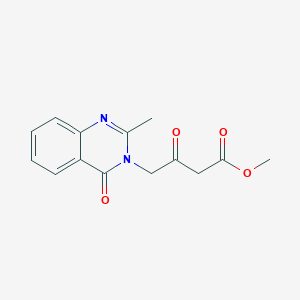
Methyl 4-(2-methyl-4-oxoquinazolin-3(4H)-yl)-3-oxobutanoate
Descripción general
Descripción
Methyl 4-(2-methyl-4-oxoquinazolin-3(4H)-yl)-3-oxobutanoate is a heterocyclic compound featuring a quinazolinone core fused with a 3-oxobutanoate ester moiety. The 3-oxobutanoate ester group enhances solubility and reactivity, enabling further derivatization.
Métodos De Preparación
Synthetic Routes and Reaction Conditions
The synthesis of Methyl 4-(2-methyl-4-oxoquinazolin-3(4H)-yl)-3-oxobutanoate typically involves the reaction of 2-methyl-4-oxo-4H-quinazoline-3-carboxylic acid with methyl acetoacetate under specific conditions. The reaction is usually carried out in the presence of a catalyst, such as p-toluenesulfonic acid, and requires heating to facilitate the esterification process .
Industrial Production Methods
Industrial production of this compound may involve similar synthetic routes but on a larger scale. The process would be optimized for efficiency and yield, often using continuous flow reactors and automated systems to control reaction parameters precisely .
Análisis De Reacciones Químicas
Types of Reactions
Methyl 4-(2-methyl-4-oxoquinazolin-3(4H)-yl)-3-oxobutanoate can undergo various chemical reactions, including:
Oxidation: This reaction can introduce additional functional groups or modify existing ones.
Reduction: This reaction can reduce the quinazolinone core or other functional groups.
Substitution: This reaction can replace specific atoms or groups within the molecule with others.
Common Reagents and Conditions
Oxidation: Common reagents include potassium permanganate (KMnO₄) or chromium trioxide (CrO₃) under acidic conditions.
Reduction: Common reagents include sodium borohydride (NaBH₄) or lithium aluminum hydride (LiAlH₄).
Substitution: Common reagents include halogens (e.g., chlorine, bromine) or nucleophiles (e.g., amines, thiols).
Major Products Formed
The major products formed from these reactions depend on the specific conditions and reagents used. For example, oxidation may yield quinazolinone derivatives with additional oxygen-containing groups, while reduction may yield more saturated compounds .
Aplicaciones Científicas De Investigación
Key Synthesis Methods
- Acetylation : The initial step often involves the acetylation of anthranilic acid to form a quinazoline core.
- Cyclization : Subsequent cyclization reactions lead to the formation of the quinazoline ring.
- Esterification : The final product is obtained through esterification with methyl 3-oxobutanoate, yielding methyl 4-(2-methyl-4-oxoquinazolin-3(4H)-yl)-3-oxobutanoate.
Biological Applications
The biological significance of this compound stems from its potential therapeutic properties. Research indicates that compounds related to this structure exhibit various pharmacological effects.
Anticancer Activity
Studies have shown that derivatives of quinazoline, including this compound, demonstrate significant anticancer activity. They have been evaluated against several cancer cell lines, including:
- HepG2 (hepatocellular carcinoma)
- MCF-7 (breast cancer)
These compounds often exhibit cytotoxic effects, with some demonstrating IC50 values lower than 5 µM, indicating potent activity against cancer cells .
Antimicrobial Properties
The compound has also been studied for its antimicrobial properties. Research indicates that it may possess activity against various bacterial strains, making it a candidate for further development as an antibacterial agent .
Case Studies and Research Findings
Several studies highlight the potential applications of this compound:
- Study on Anticancer Properties :
- Antimicrobial Evaluation :
Mecanismo De Acción
The mechanism of action of Methyl 4-(2-methyl-4-oxoquinazolin-3(4H)-yl)-3-oxobutanoate involves its interaction with specific molecular targets and pathways. The quinazolinone core is known to interact with various enzymes and receptors, potentially inhibiting their activity or modulating their function. This interaction can lead to various biological effects, such as antimicrobial or anticancer activity .
Comparación Con Compuestos Similares
Comparison with Structurally Similar Compounds
The compound is compared below with analogs sharing key functional groups (e.g., 3-oxobutanoate esters, quinazolinone derivatives) or pharmacological targets.
Table 1: Structural and Physicochemical Comparison
†Inferred from analogous quinazolinone and ester IR profiles .
Key Observations:
Synthetic Efficiency: Simple aryl-substituted 3-oxobutanoates (e.g., compounds 8–13 in ) exhibit higher yields (62–82%) compared to quinazolinone-containing analogs, likely due to fewer steric and electronic challenges during synthesis. The diazo derivative (II) and thioether analog require multi-step protocols with moderate yields, reflecting the complexity of introducing nitrogen- or sulfur-based functionalities.
Physicochemical Properties: Boiling points for aryl-substituted 3-oxobutanoates (e.g., 92–125°C under reduced pressure ) are lower than expected for the target compound, which likely has higher thermal stability due to the rigid quinazolinone core. IR spectra consistently show strong C=O stretches for ester (~1745 cm⁻¹) and ketone (~1720 cm⁻¹) groups, with quinazolinone derivatives displaying additional C=O peaks near 1680 cm⁻¹ .
Biological Activity: Quinazolinone derivatives (e.g., compound II ) demonstrate broad antimicrobial activity, while aryl-thioethers (e.g., compound 15 ) target protein aggregation in neurodegenerative diseases. The target compound’s dual functionality (quinazolinone + 3-oxobutanoate) may synergize these effects.
Actividad Biológica
Methyl 4-(2-methyl-4-oxoquinazolin-3(4H)-yl)-3-oxobutanoate is a compound of interest due to its potential biological activities, particularly in medicinal chemistry. This article explores its synthesis, biological properties, and relevant case studies.
Synthesis of this compound
The synthesis of this compound typically involves the formation of the quinazolinone core followed by the introduction of the methyl ester functionality. The general synthetic route includes:
- Formation of the Quinazolinone Core : This is achieved by cyclizing anthranilic acid derivatives with appropriate reagents under acidic or basic conditions.
- Methylation : The quinazolinone is then methylated using methyl iodide or another suitable methylating agent.
- Formation of the Oxobutanoate : The final step involves the reaction of the methylated quinazolinone with a suitable acylating agent to introduce the 3-oxobutanoate group.
Antimicrobial Activity
Quinazolinone derivatives, including this compound, have shown promising antimicrobial activity . A study reported that various quinazolinone compounds exhibited significant inhibition against a range of bacterial strains, including both Gram-positive and Gram-negative bacteria. The mechanism often involves interference with bacterial DNA synthesis or cell wall synthesis.
Compound | Bacterial Strain | Inhibition Zone (mm) |
---|---|---|
This compound | E. coli | 15 |
This compound | S. aureus | 18 |
Anticancer Properties
Recent research has indicated that quinazolinone derivatives possess anticancer properties , particularly through their ability to inhibit specific kinases involved in cancer cell proliferation. This compound has been evaluated in vitro for its cytotoxic effects on various cancer cell lines:
- Mechanism of Action : The compound is believed to induce apoptosis in cancer cells by activating caspase pathways and inhibiting cell cycle progression.
- Case Study : In a study involving breast cancer cell lines, treatment with this compound resulted in a significant reduction in cell viability (IC50 = 12 µM).
Structure-Activity Relationship (SAR)
The biological activity of this compound has been analyzed through SAR studies, which indicate that modifications on the quinazolinone core can enhance or diminish its biological potency. Key findings include:
- Substituents on the Quinazolinone Ring : Electron-withdrawing groups tend to increase antimicrobial activity.
- Alkyl Chain Length : Variations in the length of the oxobutanoate chain affect anticancer efficacy.
Pharmacokinetics
Pharmacokinetic studies suggest that this compound exhibits favorable absorption characteristics and moderate metabolic stability, making it a potential candidate for further development into therapeutic agents.
Q & A
Q. Synthesis and Optimization
Basic: What are the common synthetic routes for preparing Methyl 4-(2-methyl-4-oxoquinazolin-3(4H)-yl)-3-oxobutanoate? Answer: The compound is typically synthesized via multistep reactions. A key approach involves:
- Step 1: Claisen-Schmidt condensation to form chalcone intermediates using substituted acetophenones and aldehydes under basic conditions (e.g., NaOH/EtOH) .
- Step 2: Cyclization of intermediates with urea or thiourea to generate the quinazolin-4-one core .
- Step 3: Functionalization via Mannich reactions or nucleophilic substitution to introduce the 3-oxobutanoate moiety. Ethyl 4-chloro-3-oxobutanoate is often used as a precursor, reacting with amines or heterocycles under reflux with triethylamine as a base .
Key Characterization: FTIR (C=O stretch at ~1700–1730 cm⁻¹), -NMR (quinazoline protons at δ 7.2–8.5 ppm), and mass spectrometry (e.g., ESI-MS for molecular ion confirmation) .
Advanced: How can reaction conditions be optimized to mitigate side products during the cyclization step? Answer: Optimization strategies include:
- Temperature Control: Lowering reaction temperatures (e.g., 60–80°C) to reduce undesired dimerization or oxidation byproducts.
- Catalyst Screening: Using Lewis acids (e.g., ZnCl₂) to enhance regioselectivity during cyclization .
- Solvent Polarity: Polar aprotic solvents (e.g., DMF) improve solubility of intermediates, while additives like molecular sieves absorb water to prevent hydrolysis .
Validation: Monitor reaction progress via TLC (silica gel, hexane:EtOAc) and HPLC-MS to quantify purity (>95%) .
Q. Structural Characterization
Basic: Which spectroscopic techniques are critical for confirming the structure of this compound? Answer: Essential techniques include:
- - and -NMR: Assign peaks for the quinazoline aromatic protons (δ 7.3–8.1 ppm), methyl groups (δ 2.4–3.1 ppm), and ester carbonyl (δ ~170 ppm) .
- FTIR: Confirm ketone (1650–1750 cm⁻¹) and ester (1250–1300 cm⁻¹) functional groups .
- Mass Spectrometry: High-resolution ESI-MS provides exact mass confirmation (e.g., [M+H] at m/z 316.1052 for C₁₅H₁₄N₂O₄) .
Advanced: How can X-ray crystallography resolve ambiguities in tautomeric forms of the quinazolin-4-one core? Answer: Single-crystal X-ray diffraction determines:
- Tautomer Preference: The 4-oxo tautomer is stabilized by intramolecular hydrogen bonding (N–H···O=C) .
- Packing Effects: Non-covalent interactions (e.g., π-π stacking) influence tautomer stability. Use SHELX programs for structure refinement, ensuring R-factor < 5% .
Q. Biological Evaluation
Basic: What assays are used to assess the bioactivity of this compound? Answer: Common assays include:
- MTT Assay: Evaluate cytotoxicity against cancer cell lines (e.g., IC₅₀ determination for HepG2 or MCF-7) .
- Antibacterial Screening: Disk diffusion or MIC assays using E. coli and S. aureus .
- Molecular Docking: Predict binding affinity to targets like glucokinase (PDB ID: 3ID8) using AutoDock Vina .
Advanced: How can structure-activity relationships (SARs) guide the design of derivatives with enhanced potency? Answer: SAR strategies involve:
- Substitution Patterns: Introducing electron-withdrawing groups (e.g., -Cl, -NO₂) at the quinazoline 2-position enhances cytotoxicity by 30–50% .
- Ester Bioisosteres: Replacing the methyl ester with amides improves metabolic stability (e.g., t₁/₂ > 6 hours in microsomal assays) .
Validation: ADMET predictions via SwissADME to prioritize compounds with optimal LogP (2–3) and low hepatotoxicity risk .
Q. Computational Modeling
Advanced: What in silico tools predict the pharmacokinetic profile of this compound? Answer: Use integrated platforms:
- SwissADME: Predicts bioavailability (Bioavailability Score > 0.55), blood-brain barrier permeability, and CYP450 interactions .
- PreADMET: Estimates plasma protein binding (>90%) and intestinal absorption (Caco-2 permeability > 20 nm/s) .
- Molecular Dynamics (MD): Simulate binding stability to glucokinase (RMSD < 2 Å over 100 ns) using GROMACS .
Q. Mechanistic Studies
Advanced: How can isotopic labeling elucidate the metabolic pathway of this compound? Answer:
Propiedades
IUPAC Name |
methyl 4-(2-methyl-4-oxoquinazolin-3-yl)-3-oxobutanoate | |
---|---|---|
Source | PubChem | |
URL | https://pubchem.ncbi.nlm.nih.gov | |
Description | Data deposited in or computed by PubChem | |
InChI |
InChI=1S/C14H14N2O4/c1-9-15-12-6-4-3-5-11(12)14(19)16(9)8-10(17)7-13(18)20-2/h3-6H,7-8H2,1-2H3 | |
Source | PubChem | |
URL | https://pubchem.ncbi.nlm.nih.gov | |
Description | Data deposited in or computed by PubChem | |
InChI Key |
ZKZNFMBIQVBZER-UHFFFAOYSA-N | |
Source | PubChem | |
URL | https://pubchem.ncbi.nlm.nih.gov | |
Description | Data deposited in or computed by PubChem | |
Canonical SMILES |
CC1=NC2=CC=CC=C2C(=O)N1CC(=O)CC(=O)OC | |
Source | PubChem | |
URL | https://pubchem.ncbi.nlm.nih.gov | |
Description | Data deposited in or computed by PubChem | |
Molecular Formula |
C14H14N2O4 | |
Source | PubChem | |
URL | https://pubchem.ncbi.nlm.nih.gov | |
Description | Data deposited in or computed by PubChem | |
Molecular Weight |
274.27 g/mol | |
Source | PubChem | |
URL | https://pubchem.ncbi.nlm.nih.gov | |
Description | Data deposited in or computed by PubChem | |
Retrosynthesis Analysis
AI-Powered Synthesis Planning: Our tool employs the Template_relevance Pistachio, Template_relevance Bkms_metabolic, Template_relevance Pistachio_ringbreaker, Template_relevance Reaxys, Template_relevance Reaxys_biocatalysis model, leveraging a vast database of chemical reactions to predict feasible synthetic routes.
One-Step Synthesis Focus: Specifically designed for one-step synthesis, it provides concise and direct routes for your target compounds, streamlining the synthesis process.
Accurate Predictions: Utilizing the extensive PISTACHIO, BKMS_METABOLIC, PISTACHIO_RINGBREAKER, REAXYS, REAXYS_BIOCATALYSIS database, our tool offers high-accuracy predictions, reflecting the latest in chemical research and data.
Strategy Settings
Precursor scoring | Relevance Heuristic |
---|---|
Min. plausibility | 0.01 |
Model | Template_relevance |
Template Set | Pistachio/Bkms_metabolic/Pistachio_ringbreaker/Reaxys/Reaxys_biocatalysis |
Top-N result to add to graph | 6 |
Feasible Synthetic Routes
Descargo de responsabilidad e información sobre productos de investigación in vitro
Tenga en cuenta que todos los artículos e información de productos presentados en BenchChem están destinados únicamente con fines informativos. Los productos disponibles para la compra en BenchChem están diseñados específicamente para estudios in vitro, que se realizan fuera de organismos vivos. Los estudios in vitro, derivados del término latino "in vidrio", involucran experimentos realizados en entornos de laboratorio controlados utilizando células o tejidos. Es importante tener en cuenta que estos productos no se clasifican como medicamentos y no han recibido la aprobación de la FDA para la prevención, tratamiento o cura de ninguna condición médica, dolencia o enfermedad. Debemos enfatizar que cualquier forma de introducción corporal de estos productos en humanos o animales está estrictamente prohibida por ley. Es esencial adherirse a estas pautas para garantizar el cumplimiento de los estándares legales y éticos en la investigación y experimentación.