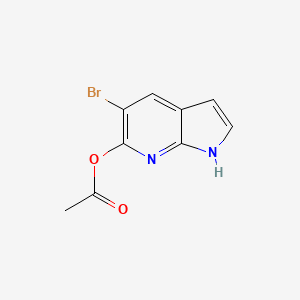
6-Acetoxy-5-bromo-7-azaindole
Descripción general
Descripción
Métodos De Preparación
Synthetic Routes and Reaction Conditions
The synthesis of 6-Acetoxy-5-bromo-7-azaindole typically involves the following steps:
Bromination: The starting material, 7-azaindole, undergoes bromination to introduce the bromine atom at the 5-position.
Acetylation: The brominated intermediate is then acetylated to introduce the acetoxy group at the 6-position.
Industrial Production Methods
Industrial production of this compound follows similar synthetic routes but may involve optimization of reaction conditions to improve yield and purity. The use of continuous flow reactors and automated synthesis platforms can enhance the efficiency and scalability of the production process .
Análisis De Reacciones Químicas
Types of Reactions
6-Acetoxy-5-bromo-7-azaindole can undergo various chemical reactions, including:
Substitution Reactions: The bromine atom at the 5-position can be substituted with other nucleophiles such as amines, thiols, or alkyl groups using palladium-catalyzed cross-coupling reactions.
Hydrolysis: The acetoxy group can be hydrolyzed to yield the corresponding hydroxyl derivative.
Oxidation and Reduction: The compound can undergo oxidation and reduction reactions to modify the functional groups and enhance its biological activity.
Common Reagents and Conditions
Palladium-catalyzed Cross-coupling: Reagents such as palladium acetate, triphenylphosphine, and base (e.g., potassium carbonate) in a suitable solvent (e.g., dimethylformamide) are commonly used.
Hydrolysis: Aqueous acid or base can be used to hydrolyze the acetoxy group.
Oxidation and Reduction: Reagents such as hydrogen peroxide for oxidation and sodium borohydride for reduction are commonly employed.
Major Products Formed
Substituted Derivatives: Various substituted derivatives can be formed depending on the nucleophile used in the substitution reactions.
Hydroxyl Derivative: Hydrolysis of the acetoxy group yields the corresponding hydroxyl derivative.
Aplicaciones Científicas De Investigación
Medicinal Chemistry
Anticancer Activity
6-Acetoxy-5-bromo-7-azaindole has been identified as a promising intermediate in the synthesis of kinase inhibitors, which are crucial in cancer therapy. Its structure allows it to interact with specific protein kinases, inhibiting their activity and consequently blocking the phosphorylation of target proteins involved in tumor progression. Research has shown that derivatives of 7-azaindoles can effectively inhibit the growth of various cancer cell lines by interfering with critical signaling pathways.
Antimicrobial Properties
The compound has also demonstrated potential antimicrobial activity. Studies indicate that certain derivatives exhibit selective activity against both Gram-positive and Gram-negative bacteria, suggesting its utility in developing new antibiotics. This is particularly relevant given the increasing resistance to existing antimicrobial agents.
Antiviral Activity
Research into azaindole derivatives, including this compound, has revealed their potential as antiviral agents. For example, compounds derived from this class have shown efficacy against HIV, making them candidates for further development in antiviral therapies .
Chemical Biology
Protein-Ligand Interaction Studies
In chemical biology, this compound serves as a valuable probe for studying protein-ligand interactions. Its ability to bind to specific molecular targets allows researchers to investigate signal transduction pathways and the molecular mechanisms underlying various biological processes.
Material Science
Development of Novel Materials
The compound's unique electronic and optical properties make it suitable for applications in material science. Research indicates that it can be utilized in developing novel materials with specific electronic characteristics, which could be beneficial in fields such as organic electronics and photonics.
Case Studies and Research Findings
-
Inhibition of Trypanosoma brucei :
A study identified a series of 3,5-disubstituted 7-azaindoles as growth inhibitors of Trypanosoma brucei, highlighting structure-activity relationships (SAR) that guide optimization for better efficacy against this pathogen. -
CDK9 Inhibition :
Research focused on azaindole derivatives as potent inhibitors of cyclin-dependent kinase 9 (CDK9), which is implicated in transcription regulation and various cancers. The study found that modifications to the azaindole core significantly influenced potency and selectivity. -
Kinase Inhibitor Development :
The unique structural features of compounds like this compound make them suitable candidates for further development into therapeutic agents targeting specific kinases involved in cancer progression.
Mecanismo De Acción
The mechanism of action of 6-Acetoxy-5-bromo-7-azaindole involves its interaction with specific molecular targets, such as protein kinases. The compound can inhibit the activity of kinases by binding to the ATP-binding site, thereby blocking the phosphorylation of target proteins . This inhibition can disrupt signal transduction pathways involved in cell growth, differentiation, and apoptosis, making it a potential therapeutic agent for various diseases .
Comparación Con Compuestos Similares
Similar Compounds
5-Bromo-7-azaindole: This compound lacks the acetoxy group but shares the bromine substitution at the 5-position.
6-Hydroxy-5-bromo-7-azaindole: This compound has a hydroxyl group instead of an acetoxy group at the 6-position.
7-Azaindole: The parent compound without any substitutions.
Uniqueness
6-Acetoxy-5-bromo-7-azaindole is unique due to the presence of both bromine and acetoxy groups, which confer distinct chemical reactivity and biological activity. The acetoxy group can be hydrolyzed to yield the hydroxyl derivative, providing versatility in chemical modifications . Additionally, the bromine atom allows for further functionalization through cross-coupling reactions, making it a valuable intermediate in synthetic chemistry .
Actividad Biológica
6-Acetoxy-5-bromo-7-azaindole is a compound that has garnered attention in medicinal chemistry due to its potential biological activities. Its unique structure, featuring both bromine and acetoxy groups, allows for various chemical reactivities that can be exploited in drug development. This article explores the biological activity of this compound, focusing on its mechanisms of action, therapeutic potential, and relevant case studies.
Chemical Structure and Properties
The molecular formula of this compound is CHBrNO, with a molecular weight of 255.07 g/mol. The presence of the bromine atom at the 5-position and the acetoxy group at the 6-position contributes to its unique chemical properties and biological interactions.
This compound acts primarily as a kinase inhibitor. It binds to the ATP-binding site of specific kinases, inhibiting their activity and thereby blocking the phosphorylation of target proteins. This mechanism is crucial in regulating various cellular processes, including cell proliferation and apoptosis.
Anticancer Activity
Research indicates that this compound exhibits significant anticancer properties. It has been used as an intermediate in synthesizing more potent kinase inhibitors targeting various cancers. For instance, studies have shown that derivatives of 7-azaindoles, including this compound, can inhibit the growth of cancer cells by interfering with key signaling pathways involved in tumor progression .
Antimicrobial Activity
The compound has also been evaluated for antimicrobial properties. In a study assessing a series of azaindole derivatives, it was noted that certain analogs demonstrated selective activity against both Gram-positive and Gram-negative bacteria. This suggests potential applications in developing new antibiotics .
Case Studies and Research Findings
- Inhibition of Trypanosoma brucei : A series of 3,5-disubstituted-7-azaindoles were identified through high-throughput screening as growth inhibitors of Trypanosoma brucei, the causative agent of human African trypanosomiasis (HAT). The study highlighted structure-activity relationships (SAR) that could guide further optimization for better efficacy against this pathogen .
- CDK9 Inhibition : Another study focused on azaindole derivatives as potent inhibitors of cyclin-dependent kinase 9 (CDK9), which plays a vital role in transcription regulation and is implicated in various cancers. The findings indicated that modifications to the azaindole core significantly influenced potency and selectivity .
- Kinase Inhibitor Development : Research has shown that azaindoles are increasingly being utilized in drug discovery for their kinase inhibition capabilities. The unique structural features of compounds like this compound make them suitable candidates for further development into therapeutic agents .
Comparative Analysis with Similar Compounds
Compound Name | Structure Features | Biological Activity |
---|---|---|
5-Bromo-7-azaindole | Lacks acetoxy group | Moderate kinase inhibition |
6-Hydroxy-5-bromo-7-azaindole | Hydroxyl group instead of acetoxy | Reduced potency |
7-Azaindole | Parent compound without substitutions | Baseline activity |
This compound stands out due to its dual functional groups, enhancing its reactivity and biological activity compared to its analogs.
Q & A
Basic Research Questions
Q. What are the optimal synthetic routes for 6-Acetoxy-5-bromo-7-azaindole, considering regioselectivity and yield?
- Methodological Answer : The synthesis typically involves palladium-catalyzed cross-coupling reactions or regioselective halogenation of the 7-azaindole core. For example, regioselective bromination can be achieved via N-oxide intermediates under controlled conditions to direct substitution at the 5-position . Subsequent acetylation at the 6-position requires anhydrous conditions and catalysts like DMAP (4-dimethylaminopyridine) to minimize side reactions. Purification via column chromatography (silica gel, ethyl acetate/hexane gradient) ensures high yield (>85%) and purity (>95%) .
Q. How is crystallographic data utilized to confirm the structure of this compound?
- Methodological Answer : Single-crystal X-ray diffraction (SC-XRD) is the gold standard for structural confirmation. Crystals grown via slow evaporation in dichloromethane/methanol (1:1) are analyzed to determine bond lengths, angles, and intermolecular interactions. Data repositories like CCDC (Cambridge Crystallographic Data Centre) provide reference codes (e.g., CCDC-2191474) for cross-verification . Discrepancies in torsional angles >5° may indicate conformational flexibility or crystal-packing effects.
Q. What spectroscopic techniques are essential for characterizing this compound?
- Methodological Answer : Nuclear Magnetic Resonance (NMR) spectroscopy (¹H, ¹³C, and 2D-COSY) resolves substituent positions, while High-Resolution Mass Spectrometry (HRMS) confirms molecular weight. For example, the 5-bromo substituent shows a characteristic downfield shift (~7.8 ppm in ¹H NMR) due to electron-withdrawing effects. Infrared (IR) spectroscopy identifies the acetyl group (C=O stretch at ~1740 cm⁻¹) .
Advanced Research Questions
Q. What strategies resolve contradictions between computational predictions and experimental reactivity data for this compound?
- Methodological Answer : Discrepancies often arise from solvent effects or incomplete basis sets in Density Functional Theory (DFT) calculations. Hybrid methods (e.g., COSMO-RS for solvation modeling) improve accuracy. For example, if DFT predicts nucleophilic attack at the 3-position but experiments show 5-position reactivity, re-evaluate solvent polarity or counterion effects in simulations . Iterative refinement using experimental kinetic data (e.g., Hammett plots) aligns computational models with observed trends .
Q. How to design factorial experiments to study substituent effects on the compound’s reactivity?
- Methodological Answer : A 2³ factorial design can systematically test variables like temperature (60°C vs. 80°C), catalyst loading (5 mol% vs. 10 mol%), and solvent polarity (THF vs. DMF). Response Surface Methodology (RSM) optimizes conditions for maximum yield or selectivity. For instance, ANOVA analysis identifies significant interactions (e.g., catalyst-solvent synergy) that dominate reaction pathways .
Q. What advanced catalytic systems improve the scalability of this compound synthesis?
- Methodological Answer : Heterogeneous catalysts (e.g., Pd/C or immobilized enzymes) enhance recyclability and reduce metal contamination. Flow chemistry setups with packed-bed reactors achieve continuous production, minimizing batch variability. Kinetic studies under flow conditions (residence time: 2–5 minutes) reveal optimal pressure and temperature profiles .
Q. How to address conflicting biological activity data in structure-activity relationship (SAR) studies?
- Methodological Answer : Contradictions may stem from assay variability (e.g., cell line differences). Standardize protocols using WHO-recommended cell lines and dose-response curves (IC₅₀ values). Molecular docking studies (e.g., AutoDock Vina) identify binding modes, while Free Energy Perturbation (FEP) calculations quantify affinity changes for SAR refinement .
Q. Data Management & Computational Approaches
Q. How can AI-driven simulations accelerate the discovery of this compound derivatives with enhanced properties?
- Methodological Answer : Generative adversarial networks (GANs) predict novel derivatives by training on existing crystallographic and reactivity datasets. COMSOL Multiphysics integrates reaction kinetics and thermodynamics to simulate multi-step syntheses virtually. Active learning algorithms prioritize high-potential candidates for experimental validation, reducing trial-and-error cycles .
Q. What protocols ensure data integrity in collaborative studies on this compound?
- Methodological Answer : Blockchain-based platforms (e.g., LabChain) timestamp raw data (NMR spectra, HPLC chromatograms) to prevent tampering. Role-based access controls restrict sensitive data (e.g., biological activity) to authorized users. Version control systems (GitLab for Science) track revisions in synthetic protocols .
Tables for Key Data
Propiedades
IUPAC Name |
(5-bromo-1H-pyrrolo[2,3-b]pyridin-6-yl) acetate | |
---|---|---|
Source | PubChem | |
URL | https://pubchem.ncbi.nlm.nih.gov | |
Description | Data deposited in or computed by PubChem | |
InChI |
InChI=1S/C9H7BrN2O2/c1-5(13)14-9-7(10)4-6-2-3-11-8(6)12-9/h2-4H,1H3,(H,11,12) | |
Source | PubChem | |
URL | https://pubchem.ncbi.nlm.nih.gov | |
Description | Data deposited in or computed by PubChem | |
InChI Key |
FGLQSSTWTLDTDJ-UHFFFAOYSA-N | |
Source | PubChem | |
URL | https://pubchem.ncbi.nlm.nih.gov | |
Description | Data deposited in or computed by PubChem | |
Canonical SMILES |
CC(=O)OC1=C(C=C2C=CNC2=N1)Br | |
Source | PubChem | |
URL | https://pubchem.ncbi.nlm.nih.gov | |
Description | Data deposited in or computed by PubChem | |
Molecular Formula |
C9H7BrN2O2 | |
Source | PubChem | |
URL | https://pubchem.ncbi.nlm.nih.gov | |
Description | Data deposited in or computed by PubChem | |
Molecular Weight |
255.07 g/mol | |
Source | PubChem | |
URL | https://pubchem.ncbi.nlm.nih.gov | |
Description | Data deposited in or computed by PubChem | |
Retrosynthesis Analysis
AI-Powered Synthesis Planning: Our tool employs the Template_relevance Pistachio, Template_relevance Bkms_metabolic, Template_relevance Pistachio_ringbreaker, Template_relevance Reaxys, Template_relevance Reaxys_biocatalysis model, leveraging a vast database of chemical reactions to predict feasible synthetic routes.
One-Step Synthesis Focus: Specifically designed for one-step synthesis, it provides concise and direct routes for your target compounds, streamlining the synthesis process.
Accurate Predictions: Utilizing the extensive PISTACHIO, BKMS_METABOLIC, PISTACHIO_RINGBREAKER, REAXYS, REAXYS_BIOCATALYSIS database, our tool offers high-accuracy predictions, reflecting the latest in chemical research and data.
Strategy Settings
Precursor scoring | Relevance Heuristic |
---|---|
Min. plausibility | 0.01 |
Model | Template_relevance |
Template Set | Pistachio/Bkms_metabolic/Pistachio_ringbreaker/Reaxys/Reaxys_biocatalysis |
Top-N result to add to graph | 6 |
Feasible Synthetic Routes
Descargo de responsabilidad e información sobre productos de investigación in vitro
Tenga en cuenta que todos los artículos e información de productos presentados en BenchChem están destinados únicamente con fines informativos. Los productos disponibles para la compra en BenchChem están diseñados específicamente para estudios in vitro, que se realizan fuera de organismos vivos. Los estudios in vitro, derivados del término latino "in vidrio", involucran experimentos realizados en entornos de laboratorio controlados utilizando células o tejidos. Es importante tener en cuenta que estos productos no se clasifican como medicamentos y no han recibido la aprobación de la FDA para la prevención, tratamiento o cura de ninguna condición médica, dolencia o enfermedad. Debemos enfatizar que cualquier forma de introducción corporal de estos productos en humanos o animales está estrictamente prohibida por ley. Es esencial adherirse a estas pautas para garantizar el cumplimiento de los estándares legales y éticos en la investigación y experimentación.