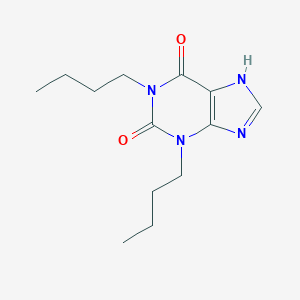
1,3-Dibutilxantina
Descripción general
Descripción
1,3-Dibutylxanthine is a xanthine derivative with the molecular formula C₁₃H₂₀N₄O₂. Xanthines are a class of alkaloids commonly found in various plants and are known for their stimulant effects on the central nervous system. 1,3-Dibutylxanthine is structurally related to other well-known xanthines such as caffeine and theophylline. This compound has been studied for its potential pharmacological properties, particularly its ability to act as an adenosine receptor antagonist .
Aplicaciones Científicas De Investigación
1,3-Dibutylxanthine has several scientific research applications, including:
Chemistry: Used as a reference compound in the study of xanthine derivatives and their chemical properties.
Biology: Investigated for its effects on adenosine receptors and potential as a biochemical tool.
Medicine: Explored for its pharmacological properties, particularly its potential as a central nervous system stimulant and adenosine receptor antagonist.
Industry: Utilized in the development of new pharmaceuticals and as a chemical intermediate in various synthetic processes
Mecanismo De Acción
Target of Action
1,3-Dibutylxanthine (DBX) is a xanthine derivative that primarily targets adenosine receptors . Adenosine receptors play a crucial role in biochemical processes like energy transfer and signal transduction. DBX binds to these receptors with high affinity and shows similar affinities for all eight adenosine receptor subtypes .
Mode of Action
DBX acts as an antagonist at the adenosine receptors . It inhibits the binding of adenosine to its receptors, thereby blocking the actions of adenosine . This interaction results in changes in cellular activity, depending on the specific function of the adenosine receptor subtype involved .
Biochemical Pathways
Adenosine is involved in a wide range of biochemical processes, including energy transfer (as adenosine triphosphate and adenosine diphosphate), signal transduction (via cyclic adenosine monophosphate), and formation of certain cofactors .
Pharmacokinetics
For instance, xanthines are generally well-absorbed from the gastrointestinal tract and widely distributed throughout the body . They are metabolized in the liver and excreted in the urine .
Result of Action
The molecular and cellular effects of DBX’s action are largely dependent on the specific adenosine receptor subtype it interacts with and the cellular context. By blocking adenosine receptors, DBX can influence a variety of physiological processes, including neurotransmission, inflammation, immune response, and cardiovascular function .
Action Environment
The action, efficacy, and stability of DBX can be influenced by various environmental factors. These may include the pH and temperature of the biological environment, the presence of other drugs or substances that can interact with adenosine receptors, and individual variations in metabolism and excretion
Análisis Bioquímico
Biochemical Properties
It is known that xanthine derivatives play a significant role in various biochemical reactions . They interact with enzymes, proteins, and other biomolecules, often serving as competitive inhibitors of various forms of phosphodiesterase, an enzyme that breaks down cyclic AMP and cyclic GMP in cells .
Cellular Effects
Xanthine derivatives are known to influence cell function, including impacts on cell signaling pathways, gene expression, and cellular metabolism .
Molecular Mechanism
Xanthine derivatives are known to exert their effects at the molecular level, including binding interactions with biomolecules, enzyme inhibition or activation, and changes in gene expression .
Dosage Effects in Animal Models
It is common for the effects of chemical compounds to vary with dosage, including potential threshold effects and toxic or adverse effects at high doses .
Metabolic Pathways
Xanthine and its derivatives are involved in purine metabolism, which includes several enzymes and cofactors .
Transport and Distribution
Many chemical compounds are transported and distributed within cells and tissues via specific transporters or binding proteins .
Subcellular Localization
The subcellular localization of a compound can affect its activity or function, and may be directed by targeting signals or post-translational modifications .
Métodos De Preparación
Synthetic Routes and Reaction Conditions
1,3-Dibutylxanthine can be synthesized through the alkylation of xanthine derivatives. One common method involves the reaction of xanthine with butyl halides in the presence of a base such as sodium hydride or potassium carbonate. The reaction typically takes place in an aprotic solvent like dimethylformamide (DMF) or dimethyl sulfoxide (DMSO) under reflux conditions .
Industrial Production Methods
Industrial production of 1,3-Dibutylxanthine may involve similar synthetic routes but on a larger scale. The process would require optimization of reaction conditions to ensure high yield and purity. This may include the use of continuous flow reactors and advanced purification techniques such as recrystallization or chromatography .
Análisis De Reacciones Químicas
Types of Reactions
1,3-Dibutylxanthine undergoes various chemical reactions, including:
Oxidation: The compound can be oxidized to form corresponding N-oxides.
Reduction: Reduction reactions can convert it into its reduced forms.
Substitution: Nucleophilic substitution reactions can introduce different substituents at the nitrogen atoms.
Common Reagents and Conditions
Oxidation: Common oxidizing agents include hydrogen peroxide and peracids.
Reduction: Reducing agents such as lithium aluminum hydride (LiAlH₄) or sodium borohydride (NaBH₄) are used.
Substitution: Alkyl halides or acyl chlorides are often used in substitution reactions.
Major Products Formed
The major products formed from these reactions depend on the specific reagents and conditions used. For example, oxidation may yield N-oxides, while substitution reactions can produce various N-alkyl or N-acyl derivatives .
Comparación Con Compuestos Similares
Similar Compounds
Caffeine (1,3,7-trimethylxanthine): A well-known stimulant found in coffee and tea.
Theophylline (1,3-dimethylxanthine): Used in the treatment of respiratory diseases like asthma.
Theobromine (3,7-dimethylxanthine): Found in cocoa and chocolate, with mild stimulant effects.
Uniqueness of 1,3-Dibutylxanthine
1,3-Dibutylxanthine is unique due to its specific substitution pattern, which imparts distinct pharmacological properties. Unlike caffeine and theophylline, which have methyl groups, 1,3-Dibutylxanthine has butyl groups, leading to differences in receptor binding affinity and metabolic stability .
Actividad Biológica
1,3-Dibutylxanthine (DBX) is a xanthine derivative that has garnered attention due to its potential biological activities, particularly in the context of adenosine receptor modulation and neuroprotective effects. This article explores the biological activity of DBX, focusing on its pharmacological properties, structure-activity relationships, and relevant case studies.
Chemical Structure and Synthesis
1,3-Dibutylxanthine is characterized by the presence of two butyl groups at the 1 and 3 positions of the xanthine backbone. Its synthesis typically involves the reaction of 5,6-diaminouracils with butyl substituents under various conditions, including microwave-assisted methods which have proven efficient in yielding high purity products .
Adenosine Receptor Affinity
DBX has been studied for its affinity towards adenosine receptors, particularly the A3 subtype. Research indicates that DBX exhibits moderate affinity for A3 receptors with a Ki value around 6 µM . The interaction with adenosine receptors is crucial as these receptors are involved in numerous physiological processes, including neurotransmission and modulation of inflammation.
Inhibition of Phosphodiesterases
Phosphodiesterase (PDE) inhibition is another significant aspect of DBX's biological activity. PDEs are enzymes that degrade cyclic nucleotides like cAMP and cGMP, thus regulating various signaling pathways. Studies have shown that DBX can inhibit certain PDEs, which may contribute to its neuroprotective effects .
Biological Activity in Neuroprotection
DBX has been evaluated for its potential neuroprotective properties. In vitro studies demonstrated that DBX could protect neuronal cells against oxidative stress-induced damage. This protective effect is hypothesized to be linked to its ability to inhibit monoamine oxidase (MAO), an enzyme associated with neurodegenerative diseases .
Table 1: Summary of Biological Activities of 1,3-Dibutylxanthine
Case Study: Neuroprotective Effects
A study investigated the neuroprotective effects of DBX on SH-SY5Y cells exposed to oxidative stress. The findings indicated that DBX significantly reduced cell death and reactive oxygen species accumulation compared to control groups. The IC50 values for MAO-B inhibition were found to be in the nanomolar range (50.7–170 nM), suggesting a strong potential for DBX as a therapeutic agent in neurodegenerative conditions .
Structure-Activity Relationship Studies
Research on structure-activity relationships (SAR) has revealed that modifications at the N1 and N3 positions can influence the biological activity of xanthines like DBX. For instance, elongation or branching of alkyl chains at these positions affects receptor affinity and enzyme inhibition potency . This information is crucial for designing new derivatives with enhanced therapeutic profiles.
Table 2: Structure-Activity Relationships for Xanthine Derivatives
Compound | N1/N3 Substituent | A3 Receptor Affinity (Ki) | MAO-B Inhibition IC50 |
---|---|---|---|
1,3-Dibutylxanthine | Butyl/Butyl | ~6 µM | 50.7 nM |
1,3-Dimethylxanthine | Methyl/Methyl | Higher affinity | Higher IC50 |
1-Ethyl-3-hexylxanthine | Ethyl/Hexyl | Lower affinity | Lower IC50 |
Propiedades
IUPAC Name |
1,3-dibutyl-7H-purine-2,6-dione | |
---|---|---|
Source | PubChem | |
URL | https://pubchem.ncbi.nlm.nih.gov | |
Description | Data deposited in or computed by PubChem | |
InChI |
InChI=1S/C13H20N4O2/c1-3-5-7-16-11-10(14-9-15-11)12(18)17(13(16)19)8-6-4-2/h9H,3-8H2,1-2H3,(H,14,15) | |
Source | PubChem | |
URL | https://pubchem.ncbi.nlm.nih.gov | |
Description | Data deposited in or computed by PubChem | |
InChI Key |
HDAPVDGMACVEKL-UHFFFAOYSA-N | |
Source | PubChem | |
URL | https://pubchem.ncbi.nlm.nih.gov | |
Description | Data deposited in or computed by PubChem | |
Canonical SMILES |
CCCCN1C2=C(C(=O)N(C1=O)CCCC)NC=N2 | |
Source | PubChem | |
URL | https://pubchem.ncbi.nlm.nih.gov | |
Description | Data deposited in or computed by PubChem | |
Molecular Formula |
C13H20N4O2 | |
Source | PubChem | |
URL | https://pubchem.ncbi.nlm.nih.gov | |
Description | Data deposited in or computed by PubChem | |
DSSTOX Substance ID |
DTXSID70182721 | |
Record name | 1,3-Dibutylxanthine | |
Source | EPA DSSTox | |
URL | https://comptox.epa.gov/dashboard/DTXSID70182721 | |
Description | DSSTox provides a high quality public chemistry resource for supporting improved predictive toxicology. | |
Molecular Weight |
264.32 g/mol | |
Source | PubChem | |
URL | https://pubchem.ncbi.nlm.nih.gov | |
Description | Data deposited in or computed by PubChem | |
CAS No. |
2850-36-4 | |
Record name | 1,3-Dibutylxanthine | |
Source | ChemIDplus | |
URL | https://pubchem.ncbi.nlm.nih.gov/substance/?source=chemidplus&sourceid=0002850364 | |
Description | ChemIDplus is a free, web search system that provides access to the structure and nomenclature authority files used for the identification of chemical substances cited in National Library of Medicine (NLM) databases, including the TOXNET system. | |
Record name | 2850-36-4 | |
Source | DTP/NCI | |
URL | https://dtp.cancer.gov/dtpstandard/servlet/dwindex?searchtype=NSC&outputformat=html&searchlist=515484 | |
Description | The NCI Development Therapeutics Program (DTP) provides services and resources to the academic and private-sector research communities worldwide to facilitate the discovery and development of new cancer therapeutic agents. | |
Explanation | Unless otherwise indicated, all text within NCI products is free of copyright and may be reused without our permission. Credit the National Cancer Institute as the source. | |
Record name | 1,3-Dibutylxanthine | |
Source | EPA DSSTox | |
URL | https://comptox.epa.gov/dashboard/DTXSID70182721 | |
Description | DSSTox provides a high quality public chemistry resource for supporting improved predictive toxicology. | |
Record name | 1,3-DIBUTYLXANTHINE | |
Source | FDA Global Substance Registration System (GSRS) | |
URL | https://gsrs.ncats.nih.gov/ginas/app/beta/substances/IR9D2UGY27 | |
Description | The FDA Global Substance Registration System (GSRS) enables the efficient and accurate exchange of information on what substances are in regulated products. Instead of relying on names, which vary across regulatory domains, countries, and regions, the GSRS knowledge base makes it possible for substances to be defined by standardized, scientific descriptions. | |
Explanation | Unless otherwise noted, the contents of the FDA website (www.fda.gov), both text and graphics, are not copyrighted. They are in the public domain and may be republished, reprinted and otherwise used freely by anyone without the need to obtain permission from FDA. Credit to the U.S. Food and Drug Administration as the source is appreciated but not required. | |
Synthesis routes and methods
Procedure details
Retrosynthesis Analysis
AI-Powered Synthesis Planning: Our tool employs the Template_relevance Pistachio, Template_relevance Bkms_metabolic, Template_relevance Pistachio_ringbreaker, Template_relevance Reaxys, Template_relevance Reaxys_biocatalysis model, leveraging a vast database of chemical reactions to predict feasible synthetic routes.
One-Step Synthesis Focus: Specifically designed for one-step synthesis, it provides concise and direct routes for your target compounds, streamlining the synthesis process.
Accurate Predictions: Utilizing the extensive PISTACHIO, BKMS_METABOLIC, PISTACHIO_RINGBREAKER, REAXYS, REAXYS_BIOCATALYSIS database, our tool offers high-accuracy predictions, reflecting the latest in chemical research and data.
Strategy Settings
Precursor scoring | Relevance Heuristic |
---|---|
Min. plausibility | 0.01 |
Model | Template_relevance |
Template Set | Pistachio/Bkms_metabolic/Pistachio_ringbreaker/Reaxys/Reaxys_biocatalysis |
Top-N result to add to graph | 6 |
Feasible Synthetic Routes
Q1: How does 1,3-Dibutylxanthine interact with adenosine receptors and what are the downstream effects?
A1: 1,3-Dibutylxanthine and its derivatives primarily interact with adenosine receptors as antagonists at A1 and A2a subtypes and as partial agonists at the A3 subtype. [, ]
- A3 Receptor Partial Agonism: As a partial agonist at A3 receptors, 1,3-dibutylxanthine can elicit a submaximal response compared to full agonists. This partial agonism has been linked to the inhibition of adenylate cyclase in some cell types, potentially influencing various cellular processes. [, ]
Q2: How does the structure of 1,3-dibutylxanthine affect its activity and selectivity for different adenosine receptor subtypes?
A2: Structure-activity relationship (SAR) studies have revealed key structural features that influence the binding affinity and selectivity of 1,3-dibutylxanthine and its derivatives for adenosine receptor subtypes:
- 1,3-Dialkyl Substituents: The length and bulkiness of the alkyl groups at positions 1 and 3 are crucial. For instance, at A3 receptors, the affinity order is pentyl ≥ butyl >> hexyl > propyl ≈ methyl, with 1,3-dipentylxanthine showing slight selectivity for A3 over A1 and A2a subtypes. []
- 8-Position Modifications: Introducing a methoxy group at position 8 is tolerated at A3 receptors. []
- 2-Position Modifications: Replacing the oxygen atom at position 2 with sulfur (2-thio vs. 2-oxo) increases potency across all three subtypes and slightly improves A3 selectivity over A1. []
- Ribose Modifications: Attaching a ribose moiety at position 7 can create xanthine-7-ribosides, some of which bind to A3 receptors. Further modifications at the ribose 5' position, such as introducing a 5'-uronamide group with varying N-alkyluronamide substituents, significantly influence A3 receptor affinity and selectivity. For example, 1,3-dibutylxanthine 7-riboside 5'-N-methylcarboxamide displays high potency (Ki = 229 nM) and significant selectivity for A3 receptors over A1 (160-fold) and A2a (>400-fold). This derivative acts as a full agonist in inhibiting adenylate cyclase via A3 receptors. []
Q3: Have any studies investigated the potential for resistance to 1,3-dibutylxanthine and its analogs in relation to other adenosine receptor ligands?
A3: While the provided research papers do not directly address resistance mechanisms specific to 1,3-dibutylxanthine, the development of resistance to G protein-coupled receptor (GPCR) ligands, including those targeting adenosine receptors, is a recognized phenomenon. [] Potential mechanisms of resistance could involve:
Descargo de responsabilidad e información sobre productos de investigación in vitro
Tenga en cuenta que todos los artículos e información de productos presentados en BenchChem están destinados únicamente con fines informativos. Los productos disponibles para la compra en BenchChem están diseñados específicamente para estudios in vitro, que se realizan fuera de organismos vivos. Los estudios in vitro, derivados del término latino "in vidrio", involucran experimentos realizados en entornos de laboratorio controlados utilizando células o tejidos. Es importante tener en cuenta que estos productos no se clasifican como medicamentos y no han recibido la aprobación de la FDA para la prevención, tratamiento o cura de ninguna condición médica, dolencia o enfermedad. Debemos enfatizar que cualquier forma de introducción corporal de estos productos en humanos o animales está estrictamente prohibida por ley. Es esencial adherirse a estas pautas para garantizar el cumplimiento de los estándares legales y éticos en la investigación y experimentación.