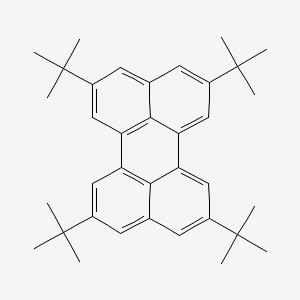
2,5,8,11-Tetra-tert-butylperylene
Descripción general
Descripción
It is a yellow crystalline solid that is insoluble in water but soluble in many organic solvents such as toluene and dimethylformamide . 2,5,8,11-Tetra-tert-butylperylene is known for its high thermal stability and high melting point, making it a valuable material in various scientific and industrial applications .
Métodos De Preparación
The preparation of 1,4,7,10-Tetra(tert-butyl)perylene is generally carried out through chemical synthetic methods. One common preparation method involves the reaction of tert-butanol with 2-bromobenzene, followed by purification of the target product through distillation . Industrial production methods often involve the acylation of tert-butyl hydroperoxide with benzoyl chloride, where a large excess of tert-butyl hydroperoxide is used, and the hydrogen chloride formed is removed in vacuo to achieve a high yield .
Análisis De Reacciones Químicas
1,4,7,10-Tetra(tert-butyl)perylene undergoes various types of chemical reactions, including oxidation, reduction, and substitution reactions. Common reagents used in these reactions include strong acids, bases, and oxidizing agents . For example, in oxidation reactions, tert-butyl hydroperoxide can be used as an oxidizing agent. The major products formed from these reactions depend on the specific conditions and reagents used, but they often include derivatives of the original compound with modified functional groups .
Aplicaciones Científicas De Investigación
Organic Light Emitting Diodes (OLEDs)
Overview : TBPe is utilized as a dopant in OLEDs due to its excellent photophysical properties. Its ability to emit blue light makes it suitable for high-performance electroluminescent devices.
Case Study : A study demonstrated the use of TBPe in an upconversion OLED that operates at an ultralow turn-on voltage of 1.47 V. The device utilized TBPe as a fluorescent dopant alongside other materials to achieve efficient charge transfer and light emission .
Property | Value |
---|---|
Turn-on Voltage | 1.47 V |
Emission Color | Blue |
Role | Fluorescent Dopant |
Photon Upconversion
Overview : TBPe plays a significant role in photon upconversion processes, where it acts as an annihilator in triplet-triplet annihilation systems. This application is crucial for enhancing the efficiency of solar cells and photonic devices.
Case Study : In a study focused on NIR-to-blue upconversion, TBPe was combined with an osmium complex to achieve an upconversion efficiency of 5.9%. The system demonstrated the capability to convert near-infrared light into blue light effectively .
Process | NIR-to-Blue Upconversion |
---|---|
Efficiency | 5.9% |
Medium | Deoxygenated DMF solution |
Application | Photocatalysis |
Photocatalysis
Overview : TBPe has been explored as a photocatalyst in various chemical reactions, particularly in the functionalization of organic compounds through energy transfer mechanisms.
Case Study : A recent study highlighted TBPe's role in facilitating Meerwein-type arylation reactions under visible light irradiation. The compound's singlet-excited state enabled the activation of aryl chlorides, leading to high product yields in subsequent reactions .
Reaction Type | Meerwein-type Arylation |
---|---|
Light Source | Visible Light |
Product Yield | High |
Mechanism | Energy Transfer |
Organic Photovoltaics (OPVs)
Overview : TBPe's electronic properties make it suitable for use in organic photovoltaics, where it can enhance charge transport and light absorption.
Case Study : Research indicated that incorporating TBPe into OPV systems improved overall efficiency by reducing molecular aggregation and enhancing exciton diffusion lengths .
Property | Value |
---|---|
Efficiency Improvement | Enhanced Charge Transport |
Mechanism | Reduced Molecular Aggregation |
Mecanismo De Acción
The mechanism by which 1,4,7,10-Tetra(tert-butyl)perylene exerts its effects is primarily through its interaction with molecular targets and pathways involved in electronic and optical processes. This steric hindrance also helps in maintaining color stability in OLEDs .
Comparación Con Compuestos Similares
1,4,7,10-Tetra(tert-butyl)perylene can be compared with other similar compounds such as perylene and its derivatives. Unlike perylene, 2,5,8,11-Tetra-tert-butylperylene has four tert-butyl groups that provide steric hindrance, reducing molecular aggregation and enhancing the efficiency of electroluminescent devices . Other similar compounds include this compound and 2,5,8,11-tetrakis(1,1-dimethylethyl)perylene, which also have tert-butyl groups but differ in their specific structural arrangements .
Actividad Biológica
2,5,8,11-Tetra-tert-butylperylene (TBPe) is a perylene derivative known for its unique photophysical properties and potential applications in various fields, including organic electronics and photochemistry. This article explores the biological activity of TBPe, emphasizing its role in photochemical processes and its interactions within biological systems.
Photophysical Properties
TBPe exhibits significant photophysical characteristics that enhance its utility in biological applications. The presence of four tert-butyl groups increases its solubility and stability compared to other perylene derivatives, which is crucial for its application in biological systems.
Table 1: Photophysical Properties of TBPe
Property | Value |
---|---|
Molecular Formula | C22H30 |
Molecular Weight | 290.48 g/mol |
Absorption Maximum | 470 nm (blue light region) |
Emission Maximum | 520 nm |
Solubility | High in organic solvents |
1. Photocatalytic Activity
Recent studies have demonstrated that TBPe can function as an efficient photocatalyst in various reactions. For instance, it has been shown to facilitate the conversion of green light to blue light through a triplet-triplet annihilation upconversion (TTA-UC) process. This capability allows TBPe to activate aryl chlorides reductively, initiating functionalization reactions such as the Mizoroki–Heck reaction and photo-Arbuzov reaction .
2. Optogenetics
TBPe has also been utilized in optogenetic applications due to its ability to act as a sensitizer in NIR-to-blue TTA-UC systems. This property enables precise control over neural activity in model organisms like Drosophila, showcasing TBPe's potential for influencing biological processes through light activation .
Case Study 1: Triplet Energy Transfer Efficiency
A study investigated the efficiency of TBPe when paired with various sensitizers for TTA-UC applications. The results indicated that TBPe could achieve a quantum yield of up to 5.9% in solid-state conditions, demonstrating its effectiveness in energy transfer processes essential for photochemical applications .
Case Study 2: Biological Response in Model Organisms
In an experimental setup involving Drosophila larvae expressing optogenetic tools, TBPe was employed to stimulate specific neural pathways. The larvae exhibited significant behavioral changes upon exposure to light activated by TBPe, indicating its potential role in modulating neural activity through optogenetics .
The biological activity of TBPe can be attributed to several mechanisms:
- Energy Transfer : TBPe facilitates energy transfer processes that are critical for various biochemical reactions.
- Reactive Species Formation : Under light exposure, TBPe can generate reactive oxygen species (ROS), which may have implications for cellular signaling and stress responses.
- Molecular Interactions : The structural characteristics of TBPe allow it to interact with biomolecules effectively, potentially influencing enzymatic activities or receptor interactions.
Q & A
Q. What are the primary applications of TBPe in optoelectronic devices, and how do its photophysical properties influence device design?
Basic Research Question
TBPe is widely used as a blue fluorescent emitter in organic light-emitting diodes (OLEDs) due to its short luminescence lifetime (~4.4 ns) and narrow emission spectrum, which reduces exciton quenching and enables high-speed electroluminescence . Its rigid molecular structure minimizes non-radiative decay, enhancing fluorescence efficiency. In device design, TBPe is typically doped into host materials like 2-methyl-9,10-bis(naphthalen-2-yl)anthracene (MADN) to balance charge transport and reduce aggregation. Methodologically, researchers use time-resolved photoluminescence (TRPL) to measure its excited-state dynamics and optimize doping concentrations (e.g., 5–10 wt%) for maximum luminance .
Q. What methods are recommended for synthesizing and purifying TBPe to achieve high purity for research applications?
Basic Research Question
TBPe synthesis typically involves alkylation of perylene precursors with tert-butyl groups under anhydrous conditions. A common purification method is sublimation (>99% purity), which removes organic impurities and ensures consistent optoelectronic performance . Researchers should monitor purity using high-performance liquid chromatography (HPLC) coupled with mass spectrometry. For reproducible results, store purified TBPe in inert atmospheres (e.g., argon) to prevent oxidation .
Q. How can researchers optimize triplet energy transfer (TET) efficiency between semiconductor nanoparticles and TBPe in hybrid systems?
Advanced Research Question
TET efficiency in systems like CdX:TBPe depends on nanoparticle size and ligand coordination density . Smaller nanoparticles (e.g., 3–5 nm) increase bandgap energy, improving TET1 efficiency, but reduce surface area for TBPe coordination. Methodologically:
-
Vary nanoparticle size via controlled colloidal synthesis.
-
Quantify Dexter-type energy transfer rates using time-resolved emission spectroscopy , noting the exponential relationship:
where is donor-acceptor distance .
-
Balance ligand density by adjusting TBPe concentration during nanoparticle functionalization .
Q. What experimental approaches are used to analyze the relationship between crystal morphology and emission properties of TBPe?
Advanced Research Question
TBPe’s emission is shape-dependent due to crystal packing effects . Key methodologies include:
- X-ray powder diffraction (XRPD) : Resolve crystal structures (e.g., cubic vs. rhombic dodecahedron morphologies) .
- Controlled crystal growth : Use solvent evaporation or hydrothermal methods to tailor morphology.
- Microspectroscopy : Correlate emission spectra (e.g., 450–500 nm) with crystal facets using scanning electron microscopy (SEM) and confocal fluorescence microscopy .
Q. How does TBPe’s molecular rigidity facilitate its use in studying vibronic coupling models?
Advanced Research Question
TBPe’s rigidity minimizes torsional vibrations, making it ideal for testing the linear vibronic coupling (LVC) Hamiltonian . Researchers validate this by:
- Simulating vibronic progression using density functional theory (DFT) with B3LYP/6-31G* basis sets.
- Comparing experimental absorption/emission spectra with computational results to quantify deviations (e.g., Huang-Rhys factors) .
- Contrasting TBPe’s behavior with flexible molecules (e.g., Maleimide) to assess LVC limitations .
Q. What strategies mitigate excimer formation in TBPe to enhance singlet fission yields?
Advanced Research Question
The steric hindrance of tert-butyl groups in TBPe reduces π-π stacking, suppressing excimer formation. Researchers confirm this via:
- Ultrafast transient absorption spectroscopy : Track singlet fission (SF) dynamics (<1 ps resolution) .
- Concentration-dependent studies : Dilute TBPe in polymer matrices (e.g., PMMA) to isolate monomeric species.
- X-ray crystallography : Confirm intermolecular distances >4 Å to prevent excimer states .
Q. How do researchers evaluate TBPe’s role in hyperfluorescence-based OLEDs using quantum dynamics simulations?
Advanced Research Question
In hyperfluorescence systems (e.g., Au-Cz/TBPe), TBPe acts as a terminal emitter. Key methodologies include:
- Quantum dynamics simulations : Calculate intersystem crossing (ISC) rates (~10 s) and Förster radii () for donor-acceptor pairs .
- Electroluminescence quantum yield (EQY) measurements : Compare simulated ISC rates with experimental EQY to validate energy harvesting efficiency .
- Temperature-dependent studies : Assess thermal stability of triplet harvesting at 77–300 K .
Q. Contradictions and Limitations
Propiedades
IUPAC Name |
2,5,8,11-tetratert-butylperylene | |
---|---|---|
Source | PubChem | |
URL | https://pubchem.ncbi.nlm.nih.gov | |
Description | Data deposited in or computed by PubChem | |
InChI |
InChI=1S/C36H44/c1-33(2,3)23-13-21-14-24(34(4,5)6)19-29-30-20-26(36(10,11)12)16-22-15-25(35(7,8)9)18-28(32(22)30)27(17-23)31(21)29/h13-20H,1-12H3 | |
Source | PubChem | |
URL | https://pubchem.ncbi.nlm.nih.gov | |
Description | Data deposited in or computed by PubChem | |
InChI Key |
BFTIPCRZWILUIY-UHFFFAOYSA-N | |
Source | PubChem | |
URL | https://pubchem.ncbi.nlm.nih.gov | |
Description | Data deposited in or computed by PubChem | |
Canonical SMILES |
CC(C)(C)C1=CC2=C3C(=C1)C=C(C=C3C4=CC(=CC5=CC(=CC2=C54)C(C)(C)C)C(C)(C)C)C(C)(C)C | |
Source | PubChem | |
URL | https://pubchem.ncbi.nlm.nih.gov | |
Description | Data deposited in or computed by PubChem | |
Molecular Formula |
C36H44 | |
Source | PubChem | |
URL | https://pubchem.ncbi.nlm.nih.gov | |
Description | Data deposited in or computed by PubChem | |
DSSTOX Substance ID |
DTXSID00567941 | |
Record name | 2,5,8,11-Tetra-tert-butylperylene | |
Source | EPA DSSTox | |
URL | https://comptox.epa.gov/dashboard/DTXSID00567941 | |
Description | DSSTox provides a high quality public chemistry resource for supporting improved predictive toxicology. | |
Molecular Weight |
476.7 g/mol | |
Source | PubChem | |
URL | https://pubchem.ncbi.nlm.nih.gov | |
Description | Data deposited in or computed by PubChem | |
CAS No. |
80663-92-9 | |
Record name | 2,5,8,11-Tetra-tert-butylperylene | |
Source | EPA DSSTox | |
URL | https://comptox.epa.gov/dashboard/DTXSID00567941 | |
Description | DSSTox provides a high quality public chemistry resource for supporting improved predictive toxicology. | |
Retrosynthesis Analysis
AI-Powered Synthesis Planning: Our tool employs the Template_relevance Pistachio, Template_relevance Bkms_metabolic, Template_relevance Pistachio_ringbreaker, Template_relevance Reaxys, Template_relevance Reaxys_biocatalysis model, leveraging a vast database of chemical reactions to predict feasible synthetic routes.
One-Step Synthesis Focus: Specifically designed for one-step synthesis, it provides concise and direct routes for your target compounds, streamlining the synthesis process.
Accurate Predictions: Utilizing the extensive PISTACHIO, BKMS_METABOLIC, PISTACHIO_RINGBREAKER, REAXYS, REAXYS_BIOCATALYSIS database, our tool offers high-accuracy predictions, reflecting the latest in chemical research and data.
Strategy Settings
Precursor scoring | Relevance Heuristic |
---|---|
Min. plausibility | 0.01 |
Model | Template_relevance |
Template Set | Pistachio/Bkms_metabolic/Pistachio_ringbreaker/Reaxys/Reaxys_biocatalysis |
Top-N result to add to graph | 6 |
Feasible Synthetic Routes
Descargo de responsabilidad e información sobre productos de investigación in vitro
Tenga en cuenta que todos los artículos e información de productos presentados en BenchChem están destinados únicamente con fines informativos. Los productos disponibles para la compra en BenchChem están diseñados específicamente para estudios in vitro, que se realizan fuera de organismos vivos. Los estudios in vitro, derivados del término latino "in vidrio", involucran experimentos realizados en entornos de laboratorio controlados utilizando células o tejidos. Es importante tener en cuenta que estos productos no se clasifican como medicamentos y no han recibido la aprobación de la FDA para la prevención, tratamiento o cura de ninguna condición médica, dolencia o enfermedad. Debemos enfatizar que cualquier forma de introducción corporal de estos productos en humanos o animales está estrictamente prohibida por ley. Es esencial adherirse a estas pautas para garantizar el cumplimiento de los estándares legales y éticos en la investigación y experimentación.