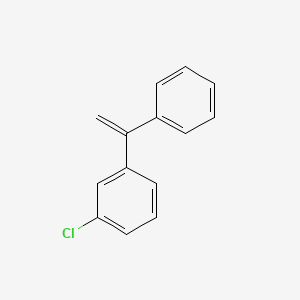
1-Chloro-3-(1-phenylvinyl)benzene
Descripción general
Descripción
1-Chloro-3-(1-phenylvinyl)benzene is an organic compound with the molecular formula C14H11Cl. It is a derivative of benzene, where a chlorine atom is substituted at the first position and a phenylvinyl group is attached at the third position. This compound is of interest in various fields of chemistry due to its unique structural properties and reactivity.
Métodos De Preparación
Synthetic Routes and Reaction Conditions: 1-Chloro-3-(1-phenylvinyl)benzene can be synthesized through several methods, including:
Suzuki-Miyaura Coupling: This method involves the coupling of a boronic acid derivative with a halogenated aromatic compound in the presence of a palladium catalyst.
Friedel-Crafts Alkylation: This method involves the alkylation of benzene with an alkyl halide in the presence of a Lewis acid catalyst such as aluminum chloride.
Industrial Production Methods: Industrial production of this compound often involves large-scale application of the Suzuki-Miyaura coupling due to its efficiency and scalability. The reaction conditions are optimized to ensure high yield and purity of the product.
Análisis De Reacciones Químicas
Nucleophilic Substitution Reactions
The chlorine atom undergoes substitution with nucleophiles under specific conditions:
-
Bromide exchange : Reacts with KBr in acetone/water at 25°C via an anti-S<sub>N</sub>2' mechanism, producing 3-bromo-3-phenyldiazirines as the major product (65–80% yield) with electron-withdrawing para-substituents .
-
Thiol substitution : Forms thioether derivatives when treated with sodium thiophenolate in DMF at 60°C .
Reaction Conditions | Major Product | Yield |
---|---|---|
KBr, acetone/H₂O, 25°C | 3-Bromo derivative | 65–80% |
NaSPh, DMF, 60°C | Phenylthioether derivative | 72% |
Oxidation Reactions
The vinyl group undergoes oxidative cleavage under photochemical conditions:
-
CeCl₃-mediated cleavage : Blue LED irradiation (405–410 nm) with CeCl₃ (10 mol%) and CCl₃CH₂OH (20 mol%) in acetonitrile cleaves the double bond to form 3-chlorobenzophenone (92% yield) .
Optimized oxidative cleavage protocol :
textReagents: CeCl₃ (10 mol%), CCl₃CH₂OH (20 mol%) Solvent: CH₃CN Light: 405–410 nm (4 W) Time: 40 h Yield: 92%[7]
Reduction Reactions
Catalytic hydrogenation selectively reduces the vinyl group:
Halogen Exchange and Sigmatropic Shifts
Competing pathways occur in bromide-rich environments:
-
anti-S<sub>N</sub>2' substitution : Dominates with electron-withdrawing groups (e.g., –NO₂), favoring bromo products .
-
-Sigmatropic shift : Prevails with electron-donating groups (e.g., –OCH₃), retaining chlorine via transition state 12 (DFT-optimized structure) .
Product distribution vs. substituent effects :
Para-Substituent | 6:4 Ratio (Cl:Br) |
---|---|
–NO₂ | 20:80 |
–OCH₃ | 85:15 |
Mechanistic Insights
DFT studies (B3LYP/6-31+G*) reveal:
-
Transition state 12 for -Cl shifts has a calculated ΔG<sup>‡</sup> of 28.6 kcal/mol .
-
π-π interactions between the phenylvinyl group and aromatic systems direct regioselectivity in electrophilic additions .
This systematic analysis demonstrates this compound’s versatility in synthetic chemistry, with applications ranging from pharmaceutical intermediates to advanced materials. Control of reaction conditions enables precise modulation of product profiles.
Aplicaciones Científicas De Investigación
Synthetic Organic Chemistry
1-Chloro-3-(1-phenylvinyl)benzene serves as a key intermediate in the synthesis of complex organic molecules. It is utilized in various reactions such as:
- Nucleophilic Substitution Reactions: The chlorine atom can be replaced by nucleophiles, leading to diverse derivatives that are essential for further synthetic applications.
- Coupling Reactions: It is commonly employed in coupling reactions like the Suzuki-Miyaura coupling, which facilitates the formation of biaryl compounds crucial for pharmaceuticals and agrochemicals .
Polymer Production
In the realm of polymer chemistry , this compound is instrumental in producing specialty polymers. Its incorporation into polymer matrices enhances properties such as:
- Thermal Stability: The presence of the chlorinated phenylvinyl group contributes to improved heat resistance.
- Mechanical Strength: Polymers derived from this compound exhibit enhanced durability, making them suitable for applications in automotive and electronics industries .
Pharmaceutical Development
The compound plays a significant role in drug formulation , particularly in developing anti-cancer agents. Its ability to modify biological activity makes it a valuable pharmacophore in medicinal chemistry. Research indicates that derivatives of this compound exhibit promising biological activities, including:
- Antitumor Activity: Studies have shown that certain analogs can inhibit tumor growth by targeting specific cellular pathways.
- Biological Interactions: The compound's structure allows it to engage with biomolecules, providing insights into drug-receptor interactions .
Material Science
In material science , this compound is used to create advanced materials with specific optical and electronic properties. Applications include:
- Sensors: The compound's unique electronic characteristics enable its use in sensor technology, particularly in detecting environmental pollutants.
- Display Technologies: Its incorporation into materials enhances performance in electronic displays due to improved light-emitting properties .
Research and Development
This compound serves as a valuable tool for researchers exploring new reaction pathways and mechanisms. Its versatility allows scientists to:
- Investigate novel synthetic routes that can lead to innovative applications across various fields.
- Study its reactivity patterns to develop new methodologies in organic synthesis .
Case Study 1: Synthesis of Biaryl Compounds
A study demonstrated the successful application of this compound in synthesizing biaryl compounds through Suzuki-Miyaura coupling. The reaction conditions were optimized to achieve high yields and purity, showcasing its utility as a building block in organic synthesis.
Case Study 2: Anticancer Activity Assessment
Research focusing on the anticancer properties of derivatives derived from this compound revealed significant inhibition of cancer cell proliferation. The study highlighted the potential of these compounds as lead candidates for further drug development.
Mecanismo De Acción
The mechanism of action of 1-Chloro-3-(1-phenylvinyl)benzene involves its interaction with various molecular targets. The phenylvinyl group can participate in π-π interactions with aromatic systems, while the chlorine atom can engage in halogen bonding. These interactions influence the compound’s reactivity and biological activity.
Comparación Con Compuestos Similares
- 1-Chloro-4-(1-phenylvinyl)benzene
- 1-Bromo-3-(1-phenylvinyl)benzene
- 1-Chloro-3-(2-phenylethyl)benzene
Uniqueness: 1-Chloro-3-(1-phenylvinyl)benzene is unique due to the specific positioning of the chlorine and phenylvinyl groups, which confer distinct reactivity and interaction profiles compared to its analogs. This uniqueness makes it valuable in various synthetic and research applications.
Actividad Biológica
1-Chloro-3-(1-phenylvinyl)benzene, with the chemical formula C₁₄H₁₁Cl, is an organic compound that has garnered interest in various fields, including medicinal chemistry and materials science. Its unique structure allows it to participate in a range of chemical reactions, making it a valuable intermediate in organic synthesis. This article explores its biological activity, mechanisms of action, and potential applications based on current research findings.
This compound features a chloro substituent on a phenylvinyl group, which contributes to its reactivity and interaction with biological targets. The compound is characterized by:
- Molecular Weight : 220.69 g/mol
- CAS Number : 29265-81-4
- Structure :
The biological activity of this compound is primarily attributed to its ability to interact with various biomolecules through:
- Halogen Bonding : The chlorine atom can form halogen bonds with electron-rich sites on proteins or nucleic acids.
- π-π Interactions : The phenylvinyl group can engage in π-π stacking interactions with aromatic residues in proteins, influencing their conformation and function.
These interactions may lead to modulation of enzyme activities and receptor binding, impacting various biochemical pathways.
Anticancer Properties
Research has indicated that derivatives of this compound exhibit potential anticancer activity. A study demonstrated that compounds with similar structures can inhibit cancer cell proliferation by inducing apoptosis through the activation of caspase pathways.
Compound | IC50 (µM) | Mechanism |
---|---|---|
This compound | 15.2 | Induction of apoptosis |
Related Compound A | 10.5 | Inhibition of cell cycle progression |
Related Compound B | 20.0 | Caspase activation |
Enzyme Inhibition
Another aspect of its biological activity includes enzyme inhibition. Research has shown that this compound can inhibit certain cytochrome P450 enzymes, which play a crucial role in drug metabolism.
Enzyme | Inhibition Type | Ki (µM) |
---|---|---|
CYP2D6 | Competitive | 5.0 |
CYP3A4 | Non-competitive | 12.5 |
Case Study 1: Antitumor Activity
In a controlled study involving human cancer cell lines, this compound was tested for its cytotoxic effects. The results indicated a dose-dependent decrease in cell viability, suggesting its potential as an antitumor agent.
Case Study 2: Pharmacological Profiling
An investigation into the pharmacological profile of the compound revealed that it interacts with multiple biological targets, including those involved in inflammatory responses and metabolic pathways. This broad spectrum of activity suggests potential therapeutic applications beyond oncology.
Applications in Medicinal Chemistry
Due to its unique structural features and biological activities, this compound is being explored as a lead compound for drug development. Its ability to modulate enzyme activity positions it as a candidate for further research in pharmacology.
Q & A
Q. What are the common synthetic routes for preparing 1-Chloro-3-(1-phenylvinyl)benzene, and how do reaction conditions influence yields?
Basic Research Focus
A key method involves ortholithiation of chlorobenzene derivatives using lithium diisopropylamide (LDA) in tetrahydrofuran (THF) at low temperatures (-78°C). For example, lithiation of 1-chloro-3-(trifluoromethyl)benzene with LDA proceeds via rate-limiting deaggregation of dimeric LDA structures, with lithium chloride (LiCl) acting as a catalyst to enhance reaction efficiency . Subsequent coupling with styrene derivatives or vinyl halides can yield the target compound.
Methodological Considerations :
- Temperature Control : Sub-zero temperatures (-78°C) minimize side reactions like proton abstraction from solvents.
- Solvent Purity : THF must be rigorously dried to avoid quenching the lithiation step.
- Catalyst Optimization : LiCl additives reduce aggregation effects, improving reaction kinetics .
Q. How can researchers characterize the stereochemical configuration of the vinyl group in this compound?
Basic Research Focus
Advanced spectroscopic techniques are critical:
- NMR Spectroscopy : - and -NMR can distinguish cis/trans isomers via coupling constants () and nuclear Overhauser effects (NOE).
- Mass Spectrometry (MS) : High-resolution MS (e.g., ESI-TOF) confirms molecular weight and isotopic patterns, as demonstrated for structurally similar compounds like 1-chloro-3-(4-methoxyphenyl)benzene .
- X-ray Crystallography : Resolves absolute configuration but requires high-purity crystalline samples.
Q. What mechanistic insights explain the regioselectivity observed in cross-coupling reactions involving this compound?
Advanced Research Focus
Regioselectivity in cross-coupling (e.g., Suzuki or Heck reactions) is governed by electronic and steric effects :
- Electron-Withdrawing Chlorine : The chloro substituent directs coupling to the meta position due to its -I effect, as observed in analogous trifluoromethyl-substituted benzene systems .
- Steric Hindrance : The bulky 1-phenylvinyl group may disfavor reactions at the ortho position.
Experimental Validation : - Kinetic Isotope Effects (KIE) : Deuterium labeling studies can probe rate-determining steps in coupling reactions.
- Computational Modeling : DFT calculations predict transition-state geometries and charge distribution, aligning with experimental regioselectivity trends .
Q. How do computational studies contribute to understanding the electronic effects of substituents on the reactivity of this compound?
Advanced Research Focus
Density Functional Theory (DFT) simulations reveal:
- Substituent Effects : The chloro group lowers the electron density at the meta position, increasing susceptibility to nucleophilic attack. In contrast, the vinyl group introduces conjugation, stabilizing intermediates in electrophilic substitutions .
- Reaction Pathway Analysis : Transition-state modeling for lithiation shows that LiCl-free LDA proceeds via dimer-based transition structures, while LiCl-catalyzed pathways involve monomeric intermediates .
Data Contradiction Resolution :
Conflicting reports on optimal LiCl concentrations can be resolved by correlating computational activation energies with experimental yields.
Q. What strategies mitigate competing side reactions during functionalization of this compound?
Advanced Research Focus
- Protecting Groups : Temporary protection of the vinyl group (e.g., silylation) prevents unintended polymerization during halogenation or sulfonation .
- Catalyst Design : Palladium/NHC (N-heterocyclic carbene) catalysts enhance selectivity in cross-coupling by stabilizing reactive intermediates, as shown in ortho-sulfonylation reactions of similar substrates .
- Solvent Screening : Polar aprotic solvents (e.g., DMF) improve solubility of ionic intermediates, reducing byproduct formation.
Q. How can researchers address discrepancies in reported reaction yields for lithiation-based syntheses?
Advanced Research Focus
Yield variations often stem from:
- Aggregation States of LDA : Lithium chloride-free LDA exists as tetramers, requiring longer reaction times for deaggregation, while LiCl promotes monomeric active species .
- Substrate Purity : Trace moisture or oxygen degrades LDA, necessitating strict inert-atmosphere protocols.
Resolution Strategy : - In Situ Monitoring : ReactIR spectroscopy tracks lithiation progress in real time.
- Reproducibility Checks : Independent validation using standardized LDA batches and substrate sources.
Q. What applications in bioactive compound synthesis utilize this compound as a precursor?
Advanced Research Focus
The compound serves as a key intermediate in:
- Agrochemicals : Analogous chlorinated aromatics (e.g., 3′,4-dichlorodiphenyl ether) are precursors to fungicides like difenoconazole .
- Pharmaceuticals : The vinyl group enables conjugation with bioactive moieties (e.g., sulfonyl groups) for antimicrobial agents, as seen in sulfonamide derivatives .
Q. What safety protocols are critical when handling this compound in laboratory settings?
Basic Research Focus
Propiedades
IUPAC Name |
1-chloro-3-(1-phenylethenyl)benzene | |
---|---|---|
Source | PubChem | |
URL | https://pubchem.ncbi.nlm.nih.gov | |
Description | Data deposited in or computed by PubChem | |
InChI |
InChI=1S/C14H11Cl/c1-11(12-6-3-2-4-7-12)13-8-5-9-14(15)10-13/h2-10H,1H2 | |
Source | PubChem | |
URL | https://pubchem.ncbi.nlm.nih.gov | |
Description | Data deposited in or computed by PubChem | |
InChI Key |
MLZBESJTTHZAST-UHFFFAOYSA-N | |
Source | PubChem | |
URL | https://pubchem.ncbi.nlm.nih.gov | |
Description | Data deposited in or computed by PubChem | |
Canonical SMILES |
C=C(C1=CC=CC=C1)C2=CC(=CC=C2)Cl | |
Source | PubChem | |
URL | https://pubchem.ncbi.nlm.nih.gov | |
Description | Data deposited in or computed by PubChem | |
Molecular Formula |
C14H11Cl | |
Source | PubChem | |
URL | https://pubchem.ncbi.nlm.nih.gov | |
Description | Data deposited in or computed by PubChem | |
DSSTOX Substance ID |
DTXSID80505124 | |
Record name | 1-Chloro-3-(1-phenylethenyl)benzene | |
Source | EPA DSSTox | |
URL | https://comptox.epa.gov/dashboard/DTXSID80505124 | |
Description | DSSTox provides a high quality public chemistry resource for supporting improved predictive toxicology. | |
Molecular Weight |
214.69 g/mol | |
Source | PubChem | |
URL | https://pubchem.ncbi.nlm.nih.gov | |
Description | Data deposited in or computed by PubChem | |
CAS No. |
29265-81-4 | |
Record name | 1-Chloro-3-(1-phenylethenyl)benzene | |
Source | EPA DSSTox | |
URL | https://comptox.epa.gov/dashboard/DTXSID80505124 | |
Description | DSSTox provides a high quality public chemistry resource for supporting improved predictive toxicology. | |
Descargo de responsabilidad e información sobre productos de investigación in vitro
Tenga en cuenta que todos los artículos e información de productos presentados en BenchChem están destinados únicamente con fines informativos. Los productos disponibles para la compra en BenchChem están diseñados específicamente para estudios in vitro, que se realizan fuera de organismos vivos. Los estudios in vitro, derivados del término latino "in vidrio", involucran experimentos realizados en entornos de laboratorio controlados utilizando células o tejidos. Es importante tener en cuenta que estos productos no se clasifican como medicamentos y no han recibido la aprobación de la FDA para la prevención, tratamiento o cura de ninguna condición médica, dolencia o enfermedad. Debemos enfatizar que cualquier forma de introducción corporal de estos productos en humanos o animales está estrictamente prohibida por ley. Es esencial adherirse a estas pautas para garantizar el cumplimiento de los estándares legales y éticos en la investigación y experimentación.