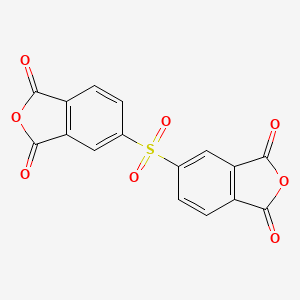
1,3-Isobenzofurandione, 5,5'-sulfonylbis-
Descripción general
Descripción
Aplicaciones Científicas De Investigación
Polyimide Synthesis
This compound is used as a raw material for synthesizing novel organosoluble polyimides and copolyimides. These materials exhibit good mechanical properties and thermal stability, making them suitable for various high-performance applications .
Aromatic Polyamide Fibers
It serves as a monomer in the synthesis of high molecular weight aromatic polyamide fibers. These fibers are known for their strength and thermal resistance, which are essential for industrial and textile applications .
High-Performance Polymer Products
As a building block, it contributes to the synthesis of polyimide films, fibers, and other polymer products that require high performance in terms of durability and stability under extreme conditions .
Hybrid Nanocomposite Films
The compound can be used in forming hybrid nanocomposite films. These films can have unique properties like enhanced electrical conductivity or improved mechanical strength, useful in electronics and materials science .
Propiedades
IUPAC Name |
5-[(1,3-dioxo-2-benzofuran-5-yl)sulfonyl]-2-benzofuran-1,3-dione | |
---|---|---|
Source | PubChem | |
URL | https://pubchem.ncbi.nlm.nih.gov | |
Description | Data deposited in or computed by PubChem | |
InChI |
InChI=1S/C16H6O8S/c17-13-9-3-1-7(5-11(9)15(19)23-13)25(21,22)8-2-4-10-12(6-8)16(20)24-14(10)18/h1-6H | |
Source | PubChem | |
URL | https://pubchem.ncbi.nlm.nih.gov | |
Description | Data deposited in or computed by PubChem | |
InChI Key |
ZHBXLZQQVCDGPA-UHFFFAOYSA-N | |
Source | PubChem | |
URL | https://pubchem.ncbi.nlm.nih.gov | |
Description | Data deposited in or computed by PubChem | |
Canonical SMILES |
C1=CC2=C(C=C1S(=O)(=O)C3=CC4=C(C=C3)C(=O)OC4=O)C(=O)OC2=O | |
Source | PubChem | |
URL | https://pubchem.ncbi.nlm.nih.gov | |
Description | Data deposited in or computed by PubChem | |
Molecular Formula |
C16H6O8S | |
Source | PubChem | |
URL | https://pubchem.ncbi.nlm.nih.gov | |
Description | Data deposited in or computed by PubChem | |
DSSTOX Substance ID |
DTXSID0073299 | |
Record name | 1,3-Isobenzofurandione, 5,5'-sulfonylbis- | |
Source | EPA DSSTox | |
URL | https://comptox.epa.gov/dashboard/DTXSID0073299 | |
Description | DSSTox provides a high quality public chemistry resource for supporting improved predictive toxicology. | |
Molecular Weight |
358.3 g/mol | |
Source | PubChem | |
URL | https://pubchem.ncbi.nlm.nih.gov | |
Description | Data deposited in or computed by PubChem | |
CAS RN |
2540-99-0 | |
Record name | 3,3′,4,4′-Diphenyl sulfone tetracarboxylic dianhydride | |
Source | CAS Common Chemistry | |
URL | https://commonchemistry.cas.org/detail?cas_rn=2540-99-0 | |
Description | CAS Common Chemistry is an open community resource for accessing chemical information. Nearly 500,000 chemical substances from CAS REGISTRY cover areas of community interest, including common and frequently regulated chemicals, and those relevant to high school and undergraduate chemistry classes. This chemical information, curated by our expert scientists, is provided in alignment with our mission as a division of the American Chemical Society. | |
Explanation | The data from CAS Common Chemistry is provided under a CC-BY-NC 4.0 license, unless otherwise stated. | |
Record name | 1,3-Isobenzofurandione, 5,5'-sulfonylbis- | |
Source | ChemIDplus | |
URL | https://pubchem.ncbi.nlm.nih.gov/substance/?source=chemidplus&sourceid=0002540990 | |
Description | ChemIDplus is a free, web search system that provides access to the structure and nomenclature authority files used for the identification of chemical substances cited in National Library of Medicine (NLM) databases, including the TOXNET system. | |
Record name | 1,3-Isobenzofurandione, 5,5'-sulfonylbis- | |
Source | EPA DSSTox | |
URL | https://comptox.epa.gov/dashboard/DTXSID0073299 | |
Description | DSSTox provides a high quality public chemistry resource for supporting improved predictive toxicology. | |
Record name | 5,5'-sulfonylbis(2-benzofuran-1,3-dione) | |
Source | European Chemicals Agency (ECHA) | |
URL | https://echa.europa.eu/substance-information/-/substanceinfo/100.234.551 | |
Description | The European Chemicals Agency (ECHA) is an agency of the European Union which is the driving force among regulatory authorities in implementing the EU's groundbreaking chemicals legislation for the benefit of human health and the environment as well as for innovation and competitiveness. | |
Explanation | Use of the information, documents and data from the ECHA website is subject to the terms and conditions of this Legal Notice, and subject to other binding limitations provided for under applicable law, the information, documents and data made available on the ECHA website may be reproduced, distributed and/or used, totally or in part, for non-commercial purposes provided that ECHA is acknowledged as the source: "Source: European Chemicals Agency, http://echa.europa.eu/". Such acknowledgement must be included in each copy of the material. ECHA permits and encourages organisations and individuals to create links to the ECHA website under the following cumulative conditions: Links can only be made to webpages that provide a link to the Legal Notice page. | |
Q & A
Q1: What is the molecular formula, weight, and key spectroscopic data for DPSDA?
A1: DPSDA has the molecular formula C20H10O10S and a molecular weight of 442.36 g/mol. Key spectroscopic data includes characteristic peaks in infrared (IR) spectroscopy corresponding to the anhydride carbonyl groups and sulfone group.
Q2: What are the solubility characteristics of DPSDA?
A2: DPSDA shows limited solubility in common organic solvents. It is typically dissolved in aprotic polar solvents like N-methyl-2-pyrrolidone (NMP) or dimethylformamide (DMF) for polymerization reactions. [, , , ]
Q3: Can DPSDA be used in the synthesis of metal-organic frameworks (MOFs)?
A4: Yes, DPSDA acts as a V-shaped linker in the formation of Al3+-based MOFs, such as CAU-11-COOH. [, ] This MOF exhibits Lewis acidity at the metal center and uncoordinated carboxyl groups, making it a promising heterogeneous catalyst.
Q4: How does CAU-11-COOH catalyze the synthesis of cyclic carbonates?
A5: CAU-11-COOH, in conjunction with a co-catalyst like tetrabutylammonium bromide (TBAB), effectively catalyzes the cycloaddition of carbon dioxide (CO₂) to epoxides, yielding cyclic carbonates under mild, solvent-free conditions. [] The Lewis acidic metal center, uncoordinated carboxyl groups, and nucleophilic bromide anion work synergistically to facilitate this reaction.
Q5: Have computational studies been performed on DPSDA-based materials?
A6: Yes, computational studies have been employed to investigate the relationship between the structure of DPSDA-derived polymers and their memory device performance. Molecular simulations revealed that electron affinity and dipole moment, influenced by the electron-withdrawing nature of DPSDA, play a crucial role in determining memory characteristics like retention time and switching voltage. []
Q6: How do structural modifications of DPSDA affect the properties of the resulting polymers?
A7: Modifying the anhydride units of DPSDA to imides can negatively impact the room-temperature phosphorescence (RTP) performance, decreasing both lifetime and quantum yield. [] The presence of multiple intermolecular interactions in the unmodified DPSDA structure is believed to restrict nonradiative decay and enhance spin-orbit coupling, leading to improved RTP properties.
Q7: Are there any alternative compounds with similar applications to DPSDA?
A8: Yes, other aromatic tetracarboxylic dianhydrides, such as pyromellitic dianhydride (PMDA), 3,3',4,4'-benzophenonetetracarboxylic dianhydride (BTDA), 4,4'-oxydiphthalic anhydride (ODPA), and 4,4'-(hexafluoroisopropylidene)diphthalic anhydride (6FDA), are commonly used in polyimide synthesis. [, , , , ] The choice of dianhydride influences the final polymer's properties, including solubility, thermal stability, and mechanical strength.
Q8: What characterization techniques are commonly employed to study DPSDA and its derivatives?
A9: Various techniques are used to characterize DPSDA and its derivatives, including:* Spectroscopy: Infrared (IR), Nuclear Magnetic Resonance (NMR) [, , ]* Thermal Analysis: Thermogravimetric analysis (TGA), Differential scanning calorimetry (DSC) [, , ]* Microscopy: Scanning electron microscopy (SEM), Atomic force microscopy (AFM) [, ]* Surface Analysis: X-ray photoelectron spectroscopy (XPS), Contact angle measurements [, ]
Q9: How has the research on DPSDA-based materials evolved?
A10: Research on DPSDA has transitioned from focusing solely on high-performance polymers like polyimides to exploring its use in diverse applications, such as gas separation membranes, [] memory devices, [, ] and heterogeneous catalysis. [, ]
Descargo de responsabilidad e información sobre productos de investigación in vitro
Tenga en cuenta que todos los artículos e información de productos presentados en BenchChem están destinados únicamente con fines informativos. Los productos disponibles para la compra en BenchChem están diseñados específicamente para estudios in vitro, que se realizan fuera de organismos vivos. Los estudios in vitro, derivados del término latino "in vidrio", involucran experimentos realizados en entornos de laboratorio controlados utilizando células o tejidos. Es importante tener en cuenta que estos productos no se clasifican como medicamentos y no han recibido la aprobación de la FDA para la prevención, tratamiento o cura de ninguna condición médica, dolencia o enfermedad. Debemos enfatizar que cualquier forma de introducción corporal de estos productos en humanos o animales está estrictamente prohibida por ley. Es esencial adherirse a estas pautas para garantizar el cumplimiento de los estándares legales y éticos en la investigación y experimentación.