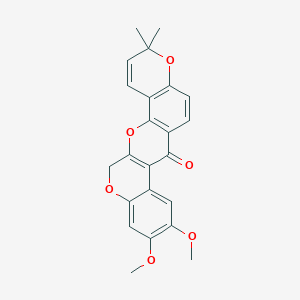
Dehidrodegüelina
Descripción general
Descripción
La dehidrodeguelina es un compuesto natural clasificado como un rotenoide, que es un tipo de flavonoide. Se deriva de plantas pertenecientes a la familia de las leguminosas, Fabaceae, como Lonchocarpus, Derris y Tephrosia . La dehidrodeguelina tiene una fórmula molecular de C23H20O6 y una masa molar de 392.4 g/mol . Es conocida por sus propiedades insecticidas y se ha estudiado por sus potenciales actividades anticancerígenas .
Aplicaciones Científicas De Investigación
Química: Se utiliza como un compuesto modelo para estudiar la química y la síntesis de rotenoides.
Biología: La dehidrodeguelina se ha investigado por sus propiedades insecticidas y sus efectos en los sistemas biológicos.
Industria: La dehidrodeguelina se utiliza en la producción de insecticidas y pesticidas naturales.
Safety and Hazards
Mecanismo De Acción
La dehidrodeguelina ejerce sus efectos a través de varios mecanismos moleculares. Induce la apoptosis (muerte celular programada) en las células cancerosas al inhibir las vías de señalización AKT/PKB. Esta inhibición está mediada a través de vías tanto dependientes de la quinasa de fosfoinositol-3-fosfato (PI3K) como independientes de PI3K . La dehidrodeguelina se dirige selectivamente a las células malignas y premalignas con efectos mínimos en las células normales .
Análisis Bioquímico
Biochemical Properties
Dehydrodeguelin plays a significant role in biochemical reactions, particularly in inhibiting cellular proliferation and inducing apoptosis in tumor cells . It interacts with various enzymes, proteins, and other biomolecules. For instance, dehydrodeguelin has been shown to inhibit the activity of the enzyme NADH:ubiquinone oxidoreductase, which is part of the mitochondrial electron transport chain . This inhibition disrupts the production of ATP, leading to increased oxidative stress and apoptosis in cancer cells. Additionally, dehydrodeguelin interacts with proteins involved in cell signaling pathways, such as the AKT/PKB signaling pathway, further contributing to its anti-cancer effects .
Cellular Effects
Dehydrodeguelin exerts various effects on different types of cells and cellular processes. In cancer cells, it induces apoptosis by activating caspases and increasing the levels of reactive oxygen species . It also inhibits cell proliferation by arresting the cell cycle at the G1 phase. Dehydrodeguelin influences cell signaling pathways, including the AKT/PKB pathway, leading to the downregulation of anti-apoptotic proteins and the upregulation of pro-apoptotic proteins . Furthermore, dehydrodeguelin affects gene expression by modulating the activity of transcription factors such as NF-κB and AP-1, which play crucial roles in cell survival and inflammation .
Molecular Mechanism
The molecular mechanism of dehydrodeguelin involves several key interactions at the molecular level. Dehydrodeguelin binds to the active site of NADH:ubiquinone oxidoreductase, inhibiting its activity and disrupting the mitochondrial electron transport chain . This leads to a decrease in ATP production and an increase in oxidative stress, ultimately resulting in apoptosis. Additionally, dehydrodeguelin inhibits the AKT/PKB signaling pathway by preventing the phosphorylation of AKT, thereby reducing the survival and proliferation of cancer cells . It also modulates gene expression by inhibiting the activity of transcription factors such as NF-κB and AP-1, which are involved in cell survival and inflammation .
Temporal Effects in Laboratory Settings
In laboratory settings, the effects of dehydrodeguelin have been observed to change over time. Dehydrodeguelin is relatively stable under standard laboratory conditions, but it can degrade over extended periods, leading to a decrease in its biological activity . Long-term studies have shown that dehydrodeguelin can have sustained effects on cellular function, including prolonged inhibition of cell proliferation and induction of apoptosis . The stability and degradation of dehydrodeguelin can vary depending on factors such as temperature, pH, and exposure to light .
Dosage Effects in Animal Models
The effects of dehydrodeguelin vary with different dosages in animal models. At low doses, dehydrodeguelin has been shown to inhibit tumor growth and induce apoptosis in cancer cells without causing significant toxicity . At higher doses, dehydrodeguelin can have toxic effects, including damage to the heart, lungs, and nervous system . Threshold effects have been observed, where the anti-cancer activity of dehydrodeguelin increases with dosage up to a certain point, beyond which toxicity becomes a concern .
Metabolic Pathways
Dehydrodeguelin is involved in several metabolic pathways, including those related to its anti-cancer activity. It interacts with enzymes such as NADH:ubiquinone oxidoreductase, which is part of the mitochondrial electron transport chain . This interaction disrupts ATP production and increases oxidative stress, leading to apoptosis in cancer cells. Dehydrodeguelin also affects metabolic flux and metabolite levels by modulating the activity of key enzymes and proteins involved in cellular metabolism .
Transport and Distribution
Dehydrodeguelin is transported and distributed within cells and tissues through various mechanisms. It can be taken up by cells via passive diffusion or active transport, depending on the concentration and cellular environment . Once inside the cell, dehydrodeguelin can interact with transporters and binding proteins that facilitate its localization and accumulation in specific cellular compartments . The distribution of dehydrodeguelin within tissues can also be influenced by factors such as blood flow, tissue permeability, and binding to plasma proteins .
Subcellular Localization
The subcellular localization of dehydrodeguelin plays a crucial role in its activity and function. Dehydrodeguelin has been found to localize primarily in the mitochondria, where it inhibits the activity of NADH:ubiquinone oxidoreductase and disrupts the mitochondrial electron transport chain . This localization is facilitated by targeting signals and post-translational modifications that direct dehydrodeguelin to the mitochondria . Additionally, dehydrodeguelin can accumulate in other cellular compartments, such as the cytoplasm and nucleus, where it can modulate gene expression and cell signaling pathways .
Métodos De Preparación
Rutas Sintéticas y Condiciones de Reacción
La síntesis de dehidrodeguelina implica la lactonización de intermedios específicos. Un enfoque incluye la lactonización directa de intermedios oxo-dehidrorotenoides . Las condiciones de reacción típicamente implican el uso de solventes orgánicos y catalizadores para facilitar la formación de la estructura del anillo lactona.
Métodos de Producción Industrial
La producción industrial de dehidrodeguelina se puede lograr mediante la extracción y purificación de fuentes vegetales. El proceso implica la extracción del material vegetal utilizando solventes, seguido de pasos de purificación como filtración y cromatografía para aislar dehidrodeguelina de alta pureza .
Análisis De Reacciones Químicas
Tipos de Reacciones
La dehidrodeguelina experimenta varias reacciones químicas, que incluyen:
Oxidación: La dehidrodeguelina se puede oxidar para formar diferentes derivados.
Reducción: Las reacciones de reducción pueden modificar los grupos funcionales presentes en la dehidrodeguelina.
Sustitución: Las reacciones de sustitución pueden introducir nuevos grupos funcionales en la molécula de dehidrodeguelina.
Reactivos y Condiciones Comunes
Los reactivos comunes utilizados en estas reacciones incluyen agentes oxidantes como el permanganato de potasio, agentes reductores como el borohidruro de sodio y varios catalizadores para facilitar las reacciones de sustitución .
Principales Productos Formados
Los principales productos formados a partir de estas reacciones dependen de los reactivos y condiciones específicas utilizadas. Por ejemplo, la oxidación puede conducir a la formación de derivados oxo-dehidrodeguelina, mientras que la reducción puede producir formas reducidas de dehidrodeguelina .
Comparación Con Compuestos Similares
La dehidrodeguelina es estructuralmente similar a otros rotenoides, como:
Deguelina: Otro rotenoide con propiedades insecticidas y anticancerígenas similares.
Alfa-Toxicarol: Un compuesto con una estructura similar pero diferentes grupos funcionales.
Tephrosina: Un rotenoide con actividades biológicas comparables.
Rotenona: Un insecticida bien conocido con una estructura estrechamente relacionada con la dehidrodeguelina.
La dehidrodeguelina es única debido a su estructura molecular específica y su actividad anticancerígena selectiva, que la distingue de otros compuestos similares .
Propiedades
IUPAC Name |
17,18-dimethoxy-7,7-dimethyl-2,8,21-trioxapentacyclo[12.8.0.03,12.04,9.015,20]docosa-1(14),3(12),4(9),5,10,15,17,19-octaen-13-one | |
---|---|---|
Source | PubChem | |
URL | https://pubchem.ncbi.nlm.nih.gov | |
Description | Data deposited in or computed by PubChem | |
InChI |
InChI=1S/C23H20O6/c1-23(2)8-7-12-15(29-23)6-5-13-21(24)20-14-9-17(25-3)18(26-4)10-16(14)27-11-19(20)28-22(12)13/h5-10H,11H2,1-4H3 | |
Source | PubChem | |
URL | https://pubchem.ncbi.nlm.nih.gov | |
Description | Data deposited in or computed by PubChem | |
InChI Key |
NGQVFILFHVPLFE-UHFFFAOYSA-N | |
Source | PubChem | |
URL | https://pubchem.ncbi.nlm.nih.gov | |
Description | Data deposited in or computed by PubChem | |
Canonical SMILES |
CC1(C=CC2=C(O1)C=CC3=C2OC4=C(C3=O)C5=CC(=C(C=C5OC4)OC)OC)C | |
Source | PubChem | |
URL | https://pubchem.ncbi.nlm.nih.gov | |
Description | Data deposited in or computed by PubChem | |
Molecular Formula |
C23H20O6 | |
Source | PubChem | |
URL | https://pubchem.ncbi.nlm.nih.gov | |
Description | Data deposited in or computed by PubChem | |
DSSTOX Substance ID |
DTXSID60188217 | |
Record name | 3H-Bis(1)benzopyrano(3,4-b:6',5'-e)pyran-7(13H)-one, 9,10-dimethoxy-3,3-dimethyl- | |
Source | EPA DSSTox | |
URL | https://comptox.epa.gov/dashboard/DTXSID60188217 | |
Description | DSSTox provides a high quality public chemistry resource for supporting improved predictive toxicology. | |
Molecular Weight |
392.4 g/mol | |
Source | PubChem | |
URL | https://pubchem.ncbi.nlm.nih.gov | |
Description | Data deposited in or computed by PubChem | |
CAS No. |
3466-23-7 | |
Record name | 3H-Bis(1)benzopyrano(3,4-b:6',5'-e)pyran-7(13H)-one, 9,10-dimethoxy-3,3-dimethyl- | |
Source | ChemIDplus | |
URL | https://pubchem.ncbi.nlm.nih.gov/substance/?source=chemidplus&sourceid=0003466237 | |
Description | ChemIDplus is a free, web search system that provides access to the structure and nomenclature authority files used for the identification of chemical substances cited in National Library of Medicine (NLM) databases, including the TOXNET system. | |
Record name | 3H-Bis(1)benzopyrano(3,4-b:6',5'-e)pyran-7(13H)-one, 9,10-dimethoxy-3,3-dimethyl- | |
Source | EPA DSSTox | |
URL | https://comptox.epa.gov/dashboard/DTXSID60188217 | |
Description | DSSTox provides a high quality public chemistry resource for supporting improved predictive toxicology. | |
Retrosynthesis Analysis
AI-Powered Synthesis Planning: Our tool employs the Template_relevance Pistachio, Template_relevance Bkms_metabolic, Template_relevance Pistachio_ringbreaker, Template_relevance Reaxys, Template_relevance Reaxys_biocatalysis model, leveraging a vast database of chemical reactions to predict feasible synthetic routes.
One-Step Synthesis Focus: Specifically designed for one-step synthesis, it provides concise and direct routes for your target compounds, streamlining the synthesis process.
Accurate Predictions: Utilizing the extensive PISTACHIO, BKMS_METABOLIC, PISTACHIO_RINGBREAKER, REAXYS, REAXYS_BIOCATALYSIS database, our tool offers high-accuracy predictions, reflecting the latest in chemical research and data.
Strategy Settings
Precursor scoring | Relevance Heuristic |
---|---|
Min. plausibility | 0.01 |
Model | Template_relevance |
Template Set | Pistachio/Bkms_metabolic/Pistachio_ringbreaker/Reaxys/Reaxys_biocatalysis |
Top-N result to add to graph | 6 |
Feasible Synthetic Routes
A: Dehydrodeguelin, along with other rotenoids, is a potent inhibitor of NADH:ubiquinone oxidoreductase, also known as Complex I, in the mitochondrial electron transport chain. [] This inhibition disrupts cellular respiration and ATP production. [, ]
ANone: Inhibition of Complex I by Dehydrodeguelin leads to a cascade of events, including:
- Depletion of ATP: Reduced electron transport hinders ATP synthesis, ultimately leading to energy depletion within the cell. [, ]
- Increased Reactive Oxygen Species (ROS): Blocking electron flow at Complex I can result in electron leakage and the generation of ROS, contributing to oxidative stress. []
- Induction of Apoptosis: The disruption of cellular energy metabolism and increased ROS can trigger programmed cell death pathways. []
A: Dehydrodeguelin has the molecular formula C23H20O6 and a molecular weight of 392.4 g/mol. [, ]
ANone: Dehydrodeguelin exhibits distinctive spectroscopic characteristics:
- UV-Vis Spectroscopy: Shows characteristic absorption maxima, useful for quantification. [] The presence of other rotenoids can interfere with UV analysis. []
- Infrared Spectroscopy (IR): Displays specific functional group vibrations, aiding in structural identification. [] Like UV analysis, the accuracy of IR analysis can be impacted by the presence of other rotenoids. []
- Nuclear Magnetic Resonance (NMR): Provides detailed structural information, including proton (1H) and carbon (13C) environments. [, , , ]
ANone: Research suggests that Dehydrodeguelin is relatively stable under various conditions:
- Light: Unlike Deguelin, Dehydrodeguelin demonstrates greater stability upon light exposure. []
- Heat and Microwave: It remains largely unaffected by heat and microwave radiation. []
ANone: Dehydrodeguelin itself is not known to possess catalytic properties. Its primary mode of action revolves around inhibiting enzymatic activity rather than catalyzing reactions.
A: While specific computational studies on Dehydrodeguelin are limited in the provided research, QSAR models have been developed for rotenoids in general. [] These models explore the relationship between chemical structure and biological activity, providing insights into the structural features influencing their potency and selectivity.
ANone: Research on Dehydrodeguelin and related rotenoids reveals that even subtle structural changes can significantly impact their activity:
- Presence of the Rotenone Moiety: The core rotenoid structure is crucial for the inhibitory activity against Complex I. []
- Substitutions on the A and B rings: Modifications to the functional groups on these rings can influence potency and selectivity towards different insect species. []
- Stereochemistry: The stereochemistry at specific positions within the molecule is crucial for activity. []
Descargo de responsabilidad e información sobre productos de investigación in vitro
Tenga en cuenta que todos los artículos e información de productos presentados en BenchChem están destinados únicamente con fines informativos. Los productos disponibles para la compra en BenchChem están diseñados específicamente para estudios in vitro, que se realizan fuera de organismos vivos. Los estudios in vitro, derivados del término latino "in vidrio", involucran experimentos realizados en entornos de laboratorio controlados utilizando células o tejidos. Es importante tener en cuenta que estos productos no se clasifican como medicamentos y no han recibido la aprobación de la FDA para la prevención, tratamiento o cura de ninguna condición médica, dolencia o enfermedad. Debemos enfatizar que cualquier forma de introducción corporal de estos productos en humanos o animales está estrictamente prohibida por ley. Es esencial adherirse a estas pautas para garantizar el cumplimiento de los estándares legales y éticos en la investigación y experimentación.