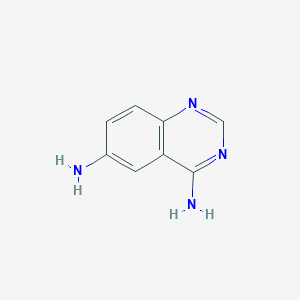
4,6-Diaminoquinazolina
Descripción general
Descripción
4,6-Quinazolinediamine, also known as 4,6-Quinazolinediamine, is a useful research compound. Its molecular formula is C8H8N4 and its molecular weight is 160.18 g/mol. The purity is usually 95%.
BenchChem offers high-quality 4,6-Quinazolinediamine suitable for many research applications. Different packaging options are available to accommodate customers' requirements. Please inquire for more information about 4,6-Quinazolinediamine including the price, delivery time, and more detailed information at info@benchchem.com.
Aplicaciones Científicas De Investigación
Actividad Anticancerígena
Se ha descubierto que las quinazolinas exhiben una actividad anticancerígena significativa . Actúan sobre diferentes dianas en el cuerpo, lo que las convierte en candidatas prometedoras para el desarrollo de nuevos fármacos contra el cáncer . Por ejemplo, un derivado específico, la 4-N-[2-(4-fenoxifenil)etil]quinazolina-4,6-diamina, se ha identificado como un potente inhibidor de una proteína de membrana multisubunidad, que podría usarse potencialmente en el tratamiento del cáncer .
Actividad Antimicrobiana y Antifúngica
Se ha descubierto que estos compuestos poseen propiedades antimicrobianas y antifúngicas . Esto las hace útiles en el tratamiento de diversas infecciones causadas por bacterias y hongos.
Actividad Antiinflamatoria
Las quinazolinas exhiben actividad antiinflamatoria . Esto sugiere que podrían utilizarse en el tratamiento de afecciones caracterizadas por inflamación, como la artritis y ciertas enfermedades autoinmunes.
Actividad Antituberculosa
Las quinazolinas han mostrado potencial en el tratamiento de la tuberculosis . Esta es una enfermedad infecciosa grave que afecta principalmente los pulmones pero también puede afectar otras partes del cuerpo.
Actividad Antimalárica
Estos compuestos han demostrado actividad antimalárica . Esto sugiere que podrían utilizarse en la prevención y el tratamiento de la malaria, una enfermedad mortal transmitida por las picaduras de mosquitos infectados.
Inhibición de Enzimas Específicas
Se ha descubierto que las quinazolinas inhiben ciertas enzimas, como la timidilato sintasa, la poli(ADP-ribosa) polimerasa (PARP) y la tirosina quinasa<a aria-label="2: " data-citationid="c850b72d-532b-0f25-8b80-b9e19dbb3ed3-38" h="
Mecanismo De Acción
Target of Action
Quinazoline-4,6-diamine, also known as 4,6-Diaminoquinazoline or 4,6-Quinazolinediamine, has been found to interact with several targets. Among the key targets of quinazoline derivatives are kinases, transcription factors, receptors, and ion channels . For instance, a synthesized compound, 4-(4-bromo-2-fluorophenylamino)-6-methoxyquinazolin-7-ol, is a potent inhibitor of vascular endothelial cell growth (VEGF) RTK, and also exhibits activity against epidermal growth factor .
Mode of Action
The compound interacts with its targets, leading to various changes. For example, it inhibits the VEGF RTK, which plays a crucial role in angiogenesis . By inhibiting this target, the compound can potentially suppress the formation of new blood vessels, which is a critical process in tumor growth and metastasis.
Biochemical Pathways
Quinazoline-4,6-diamine affects several biochemical pathways. One significant pathway is the mitochondrial complex I-dependent respiration. The compound specifically and effectively reduces this respiration with no effect on the respiratory chain complexes II-IV . This action can lead to the release of reactive oxygen species at the flavin site of mitochondrial complex I .
Pharmacokinetics
It’s known that the structure-activity relationship is critical for the observed inhibition . More research is needed to fully understand the ADME properties of this compound and their impact on its bioavailability.
Result of Action
The molecular and cellular effects of Quinazoline-4,6-diamine’s action are diverse. For instance, it has been found to reduce the level of the huntingtin protein, which could potentially make it a useful treatment for Huntington’s disease . Moreover, it has been shown to modulate calcium signaling .
Análisis Bioquímico
Biochemical Properties
Quinazoline-4,6-diamine interacts with various enzymes, proteins, and other biomolecules, contributing to its diverse biological activities. For instance, it has been found to inhibit mitochondrial complex I, a multisubunit membrane protein, reducing the mitochondrial complex I-dependent respiration . This interaction is critical for its observed inhibition .
Cellular Effects
Quinazoline-4,6-diamine has significant effects on various types of cells and cellular processes. It influences cell function by impacting cell signaling pathways, gene expression, and cellular metabolism . For example, it has been found to have acute hypotensive effects in Wistar rats, indicating its influence on vascular smooth muscle cells .
Molecular Mechanism
Quinazoline-4,6-diamine exerts its effects at the molecular level through various mechanisms. It binds to biomolecules, inhibits or activates enzymes, and induces changes in gene expression . For instance, it has been found to block NF-κB signaling at nanomolar concentrations .
Metabolic Pathways
Quinazoline-4,6-diamine is involved in various metabolic pathways. It interacts with enzymes or cofactors and can affect metabolic flux or metabolite levels . For instance, it specifically and effectively reduces the mitochondrial complex I-dependent respiration .
Actividad Biológica
4,6-Quinazolinediamine, also known as 4,6-diaminoquinazoline, is a heterocyclic compound that has garnered significant attention in medicinal chemistry due to its diverse biological activities. This article explores the biological activity of 4,6-quinazolinediamine, focusing on its mechanisms of action, pharmacological properties, and potential therapeutic applications.
Chemical Structure and Properties
The molecular formula of 4,6-quinazolinediamine is , characterized by a quinazoline ring with amino groups at the 4 and 6 positions. This unique structure allows for various chemical modifications that enhance its biological activity.
Target Interactions
4,6-Quinazolinediamine interacts with several biological targets, including:
- Tyrosine Kinases : It selectively inhibits various tyrosine kinases involved in cell growth and differentiation. For instance, derivatives like Arry-380 target HER2, a receptor overexpressed in certain breast cancers .
- VEGF Receptor Tyrosine Kinase (RTK) : The compound inhibits VEGF RTK, crucial for angiogenesis.
- Mitochondrial Complex I : It reduces mitochondrial complex I-dependent respiration without affecting other respiratory chain complexes.
Anticancer Properties
Numerous studies have demonstrated the anticancer potential of 4,6-quinazolinediamine and its derivatives:
- Inhibition of Cancer Cell Proliferation : Compounds derived from this scaffold have shown efficacy against various cancer cell lines by inhibiting key signaling pathways. For example, certain derivatives exhibit IC50 values as low as against specific targets .
- Mechanisms of Action : The anticancer activity is attributed to the inhibition of multiple molecular targets including epidermal growth factor receptor (EGFR) and vascular endothelial growth factor receptor (VEGFR) pathways .
Antibacterial Activity
Research indicates that 4,6-quinazolinediamine derivatives possess antibacterial properties:
- Effectiveness Against Resistant Strains : These compounds have shown activity against methicillin-resistant Staphylococcus aureus (MRSA) and Escherichia coli, highlighting their potential as novel antibiotics in the face of rising antibiotic resistance.
Case Studies and Research Findings
Several studies underscore the therapeutic potential of 4,6-quinazolinediamine:
- Anticancer Activity : A study evaluated a series of 2-substituted quinazoline derivatives for their ability to inhibit topoisomerase I and II in various cancer cell lines. The results indicated significant cytotoxic effects with IC50 values ranging from to .
- Inhibitory Effects on Tyrosine Kinases : Another investigation focused on the structure-activity relationship (SAR) of quinazoline derivatives, revealing that substitutions at specific positions significantly influence their inhibitory potency against tyrosine kinases .
- Pharmacokinetics : The pharmacokinetic profiles of these compounds are being explored to understand their absorption, distribution, metabolism, and excretion (ADME) properties better. Initial findings suggest favorable profiles that warrant further investigation for clinical applications.
Summary Table of Biological Activities
Activity Type | Example Derivatives | Mechanism | IC50 Value |
---|---|---|---|
Anticancer | Arry-380 | HER2 inhibition | |
Antibacterial | Various derivatives | Inhibition of bacterial growth | Varies by strain |
Angiogenesis Inhibition | Quinazoline analogs | VEGF RTK inhibition | Not specified |
Topoisomerase Inhibition | 2-substituted derivatives | Inhibition in cancer cells |
Propiedades
IUPAC Name |
quinazoline-4,6-diamine | |
---|---|---|
Source | PubChem | |
URL | https://pubchem.ncbi.nlm.nih.gov | |
Description | Data deposited in or computed by PubChem | |
InChI |
InChI=1S/C8H8N4/c9-5-1-2-7-6(3-5)8(10)12-4-11-7/h1-4H,9H2,(H2,10,11,12) | |
Source | PubChem | |
URL | https://pubchem.ncbi.nlm.nih.gov | |
Description | Data deposited in or computed by PubChem | |
InChI Key |
RNWDENXDCQXZLH-UHFFFAOYSA-N | |
Source | PubChem | |
URL | https://pubchem.ncbi.nlm.nih.gov | |
Description | Data deposited in or computed by PubChem | |
Canonical SMILES |
C1=CC2=C(C=C1N)C(=NC=N2)N | |
Source | PubChem | |
URL | https://pubchem.ncbi.nlm.nih.gov | |
Description | Data deposited in or computed by PubChem | |
Molecular Formula |
C8H8N4 | |
Source | PubChem | |
URL | https://pubchem.ncbi.nlm.nih.gov | |
Description | Data deposited in or computed by PubChem | |
DSSTOX Substance ID |
DTXSID70401020 | |
Record name | 4,6-Quinazolinediamine | |
Source | EPA DSSTox | |
URL | https://comptox.epa.gov/dashboard/DTXSID70401020 | |
Description | DSSTox provides a high quality public chemistry resource for supporting improved predictive toxicology. | |
Molecular Weight |
160.18 g/mol | |
Source | PubChem | |
URL | https://pubchem.ncbi.nlm.nih.gov | |
Description | Data deposited in or computed by PubChem | |
CAS No. |
159382-23-7 | |
Record name | 4,6-Quinazolinediamine | |
Source | EPA DSSTox | |
URL | https://comptox.epa.gov/dashboard/DTXSID70401020 | |
Description | DSSTox provides a high quality public chemistry resource for supporting improved predictive toxicology. | |
Retrosynthesis Analysis
AI-Powered Synthesis Planning: Our tool employs the Template_relevance Pistachio, Template_relevance Bkms_metabolic, Template_relevance Pistachio_ringbreaker, Template_relevance Reaxys, Template_relevance Reaxys_biocatalysis model, leveraging a vast database of chemical reactions to predict feasible synthetic routes.
One-Step Synthesis Focus: Specifically designed for one-step synthesis, it provides concise and direct routes for your target compounds, streamlining the synthesis process.
Accurate Predictions: Utilizing the extensive PISTACHIO, BKMS_METABOLIC, PISTACHIO_RINGBREAKER, REAXYS, REAXYS_BIOCATALYSIS database, our tool offers high-accuracy predictions, reflecting the latest in chemical research and data.
Strategy Settings
Precursor scoring | Relevance Heuristic |
---|---|
Min. plausibility | 0.01 |
Model | Template_relevance |
Template Set | Pistachio/Bkms_metabolic/Pistachio_ringbreaker/Reaxys/Reaxys_biocatalysis |
Top-N result to add to graph | 6 |
Feasible Synthetic Routes
Descargo de responsabilidad e información sobre productos de investigación in vitro
Tenga en cuenta que todos los artículos e información de productos presentados en BenchChem están destinados únicamente con fines informativos. Los productos disponibles para la compra en BenchChem están diseñados específicamente para estudios in vitro, que se realizan fuera de organismos vivos. Los estudios in vitro, derivados del término latino "in vidrio", involucran experimentos realizados en entornos de laboratorio controlados utilizando células o tejidos. Es importante tener en cuenta que estos productos no se clasifican como medicamentos y no han recibido la aprobación de la FDA para la prevención, tratamiento o cura de ninguna condición médica, dolencia o enfermedad. Debemos enfatizar que cualquier forma de introducción corporal de estos productos en humanos o animales está estrictamente prohibida por ley. Es esencial adherirse a estas pautas para garantizar el cumplimiento de los estándares legales y éticos en la investigación y experimentación.