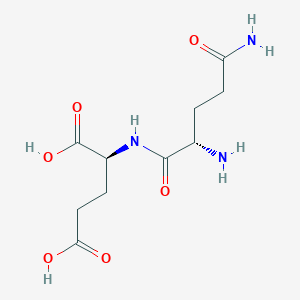
Gln-Glu
Descripción general
Descripción
Glutamine is a conditionally essential amino acid, meaning the body can usually synthesize sufficient amounts, but in some instances of stress, the body’s demand for glutamine increases, and it must be obtained from the diet . Glutamate, on the other hand, is a non-essential amino acid that plays a crucial role in cellular metabolism and neurotransmission .
Mecanismo De Acción
Target of Action
The primary targets of H-GLN-GLU-OH, also known as Gln-Glu, are the enzymes involved in the glutamate-glutamine cycle, such as glutamine synthetase (GS) and phosphate-activated glutaminase (PAG) . These enzymes play a crucial role in the conversion of glutamate (Glu) to glutamine (Gln) and vice versa .
Mode of Action
H-GLN-GLU-OH interacts with its targets by participating in the glutamate-glutamine cycle. In this cycle, glutamine synthetase, located in astrocytes, converts glutamate and ammonia into glutamine . This glutamine is then released into the extracellular space and taken up by neurons . Within the neurons, phosphate-activated glutaminase converts glutamine back into glutamate, which can then be used in neurotransmission .
Biochemical Pathways
H-GLN-GLU-OH affects several biochemical pathways. It plays a key role in the glutamate-glutamine cycle, which is crucial for glutamatergic neurotransmission . Additionally, it is involved in the synthesis of other amino acids, nucleotides, amino sugars, and vitamin B coenzymes . It also participates in the TCA cycle, providing an entry point for inorganic nitrogen .
Pharmacokinetics
Glutamine, one of the components of h-gln-glu-oh, is known to be well-absorbed and distributed throughout the body, including the brain . It is metabolized in various tissues, particularly the liver, and excreted primarily as ammonia in the urine .
Result of Action
The action of H-GLN-GLU-OH results in several molecular and cellular effects. It contributes to the regulation of glutamatergic neurotransmission, which is crucial for normal brain activity . It also plays a role in maintaining glutamine homeostasis in the brain, which is important for emotional and cognitive functions . Moreover, it has been implicated in activating stress and defense responses in plants .
Action Environment
The action, efficacy, and stability of H-GLN-GLU-OH can be influenced by various environmental factors. For instance, the availability of other nutrients, such as glucose, can affect the utilization of glutamine . Additionally, the presence of reactive oxygen species can alter glutamine metabolism, leading to changes in cell growth and survival . Furthermore, the pH and temperature of the environment can affect the activity of the enzymes involved in the glutamate-glutamine cycle .
Análisis Bioquímico
Biochemical Properties
Glutamine-Glutamic Acid (Gln-Glu) is involved in several biochemical reactions. Glutamine serves as a precursor for the synthesis of glutamic acid through the action of the enzyme glutaminase . Glutamic acid, in turn, can be converted to alpha-ketoglutarate by glutamate dehydrogenase or transaminase reactions, entering the tricarboxylic acid (TCA) cycle . Additionally, Glutamine-Glutamic Acid (this compound) interacts with various biomolecules, including asparagine synthetase, which converts glutamine to asparagine . These interactions highlight the importance of Glutamine-Glutamic Acid (this compound) in maintaining cellular homeostasis and energy production.
Cellular Effects
Glutamine-Glutamic Acid (this compound) influences various cellular processes. It plays a crucial role in cell signaling pathways, particularly in the regulation of mTOR (mechanistic target of rapamycin) signaling, which is essential for cell growth and proliferation . Glutamine-Glutamic Acid (this compound) also affects gene expression by modulating the activity of transcription factors such as c-Myc . Furthermore, it impacts cellular metabolism by serving as a source of carbon and nitrogen for the synthesis of nucleotides, amino acids, and other biomolecules .
Molecular Mechanism
At the molecular level, Glutamine-Glutamic Acid (this compound) exerts its effects through various binding interactions and enzymatic reactions. Glutamine is transported into cells via specific transporters such as ASCT2 and SNAT2 . Once inside the cell, glutamine is converted to glutamic acid by glutaminase, releasing ammonia in the process . Glutamic acid can then be further metabolized to alpha-ketoglutarate, which enters the TCA cycle . These reactions are tightly regulated by enzymes and cofactors, ensuring the proper functioning of cellular metabolism.
Temporal Effects in Laboratory Settings
In laboratory settings, the effects of Glutamine-Glutamic Acid (this compound) can change over time. The stability and degradation of Glutamine-Glutamic Acid (this compound) are influenced by factors such as temperature, pH, and the presence of other biomolecules . Long-term studies have shown that Glutamine-Glutamic Acid (this compound) can impact cellular function by modulating gene expression and metabolic pathways . These temporal effects are crucial for understanding the role of Glutamine-Glutamic Acid (this compound) in various biological processes.
Dosage Effects in Animal Models
The effects of Glutamine-Glutamic Acid (this compound) vary with different dosages in animal models. Low doses of Glutamine-Glutamic Acid (this compound) have been shown to promote cell growth and proliferation, while high doses can lead to toxic effects and cell death . Threshold effects have been observed, where a certain concentration of Glutamine-Glutamic Acid (this compound) is required to elicit a biological response . These dosage effects are important for determining the optimal concentration of Glutamine-Glutamic Acid (this compound) for therapeutic applications.
Metabolic Pathways
Glutamine-Glutamic Acid (this compound) is involved in several metabolic pathways. Glutamine serves as a precursor for the synthesis of glutamic acid, which can be further converted to alpha-ketoglutarate and enter the TCA cycle . Additionally, Glutamine-Glutamic Acid (this compound) is involved in the synthesis of nucleotides, amino acids, and other biomolecules . These metabolic pathways are essential for maintaining cellular homeostasis and energy production.
Transport and Distribution
Glutamine-Glutamic Acid (this compound) is transported and distributed within cells and tissues through specific transporters and binding proteins. Glutamine is transported into cells via ASCT2 and SNAT2 transporters, while glutamic acid is transported by EAAT (excitatory amino acid transporter) proteins . These transporters ensure the proper localization and accumulation of Glutamine-Glutamic Acid (this compound) within cells, allowing it to exert its biological effects.
Subcellular Localization
The subcellular localization of Glutamine-Glutamic Acid (this compound) is crucial for its activity and function. Glutamine is primarily localized in the cytoplasm, where it is converted to glutamic acid by glutaminase . Glutamic acid can then be transported into the mitochondria, where it is further metabolized to alpha-ketoglutarate and enters the TCA cycle . These localization patterns are essential for understanding the role of Glutamine-Glutamic Acid (this compound) in cellular metabolism and energy production.
Métodos De Preparación
Synthetic Routes and Reaction Conditions: The synthesis of Gln-Glu typically involves the enzymatic or chemical coupling of glutamine and glutamate. One common method is the use of glutaminase to convert glutamine to glutamate, followed by the coupling of the two amino acids using peptide bond formation techniques . This process often requires specific reaction conditions, such as controlled pH and temperature, to ensure the stability and activity of the enzymes involved.
Industrial Production Methods: In industrial settings, the production of this compound can be scaled up using bioreactors where microbial fermentation is employed to produce large quantities of glutamine and glutamate. These amino acids are then purified and chemically coupled to form the dipeptide. The use of genetically engineered microorganisms can enhance the yield and efficiency of this process .
Análisis De Reacciones Químicas
Types of Reactions: Gln-Glu undergoes various chemical reactions, including deamidation, oxidation, and hydrolysis. Deamidation is a common reaction where the amide group of glutamine is converted to a carboxyl group, resulting in the formation of glutamate . Oxidation reactions can occur under specific conditions, leading to the formation of reactive oxygen species and subsequent degradation of the dipeptide .
Common Reagents and Conditions: Common reagents used in the reactions involving this compound include oxidizing agents like hydrogen peroxide and reducing agents such as dithiothreitol. Enzymatic reactions often require cofactors like NAD+ and specific buffers to maintain the optimal pH .
Major Products Formed: The major products formed from the reactions of this compound include glutamate, pyroglutamic acid, and various oxidative degradation products. These products can be analyzed using techniques like liquid chromatography-mass spectrometry (LC-MS) to determine their structure and concentration .
Aplicaciones Científicas De Investigación
Chemistry: In chemistry, Gln-Glu is used as a model compound to study peptide bond formation and hydrolysis. It is also employed in the development of analytical methods for amino acid quantification .
Biology: In biological research, this compound plays a significant role in studying cellular metabolism and signaling pathways. It is used to investigate the glutamate-glutamine cycle, which is crucial for neurotransmitter recycling in the brain .
Medicine: In medicine, this compound is explored for its potential therapeutic applications. Glutamine supplementation has been shown to improve outcomes in patients with hypercatabolic conditions, while glutamate is studied for its role in neurodegenerative diseases .
Industry: In the industrial sector, this compound is used in the production of nutritional supplements and as a component in cell culture media for biopharmaceutical production .
Comparación Con Compuestos Similares
Similar Compounds: Similar compounds to Gln-Glu include other dipeptides like Ala-Gln (alanine-glutamine) and Gly-Glu (glycine-glutamate). These dipeptides share similar structural features but differ in their amino acid composition and specific biological functions .
Uniqueness: this compound is unique due to its dual role in both metabolic and neurotransmitter pathways. While other dipeptides may participate in metabolic processes, this compound’s involvement in the glutamate-glutamine cycle sets it apart as a critical component in neurotransmission and cellular signaling .
Propiedades
IUPAC Name |
(2S)-2-[[(2S)-2,5-diamino-5-oxopentanoyl]amino]pentanedioic acid | |
---|---|---|
Source | PubChem | |
URL | https://pubchem.ncbi.nlm.nih.gov | |
Description | Data deposited in or computed by PubChem | |
InChI |
InChI=1S/C10H17N3O6/c11-5(1-3-7(12)14)9(17)13-6(10(18)19)2-4-8(15)16/h5-6H,1-4,11H2,(H2,12,14)(H,13,17)(H,15,16)(H,18,19)/t5-,6-/m0/s1 | |
Source | PubChem | |
URL | https://pubchem.ncbi.nlm.nih.gov | |
Description | Data deposited in or computed by PubChem | |
InChI Key |
OWOFCNWTMWOOJJ-WDSKDSINSA-N | |
Source | PubChem | |
URL | https://pubchem.ncbi.nlm.nih.gov | |
Description | Data deposited in or computed by PubChem | |
Canonical SMILES |
C(CC(=O)N)C(C(=O)NC(CCC(=O)O)C(=O)O)N | |
Source | PubChem | |
URL | https://pubchem.ncbi.nlm.nih.gov | |
Description | Data deposited in or computed by PubChem | |
Isomeric SMILES |
C(CC(=O)N)[C@@H](C(=O)N[C@@H](CCC(=O)O)C(=O)O)N | |
Source | PubChem | |
URL | https://pubchem.ncbi.nlm.nih.gov | |
Description | Data deposited in or computed by PubChem | |
Molecular Formula |
C10H17N3O6 | |
Source | PubChem | |
URL | https://pubchem.ncbi.nlm.nih.gov | |
Description | Data deposited in or computed by PubChem | |
DSSTOX Substance ID |
DTXSID501309175 | |
Record name | L-Glutaminyl-L-glutamic acid | |
Source | EPA DSSTox | |
URL | https://comptox.epa.gov/dashboard/DTXSID501309175 | |
Description | DSSTox provides a high quality public chemistry resource for supporting improved predictive toxicology. | |
Molecular Weight |
275.26 g/mol | |
Source | PubChem | |
URL | https://pubchem.ncbi.nlm.nih.gov | |
Description | Data deposited in or computed by PubChem | |
Physical Description |
Solid | |
Record name | Glutaminylglutamic acid | |
Source | Human Metabolome Database (HMDB) | |
URL | http://www.hmdb.ca/metabolites/HMDB0028796 | |
Description | The Human Metabolome Database (HMDB) is a freely available electronic database containing detailed information about small molecule metabolites found in the human body. | |
Explanation | HMDB is offered to the public as a freely available resource. Use and re-distribution of the data, in whole or in part, for commercial purposes requires explicit permission of the authors and explicit acknowledgment of the source material (HMDB) and the original publication (see the HMDB citing page). We ask that users who download significant portions of the database cite the HMDB paper in any resulting publications. | |
CAS No. |
88830-90-4 | |
Record name | L-Glutaminyl-L-glutamic acid | |
Source | CAS Common Chemistry | |
URL | https://commonchemistry.cas.org/detail?cas_rn=88830-90-4 | |
Description | CAS Common Chemistry is an open community resource for accessing chemical information. Nearly 500,000 chemical substances from CAS REGISTRY cover areas of community interest, including common and frequently regulated chemicals, and those relevant to high school and undergraduate chemistry classes. This chemical information, curated by our expert scientists, is provided in alignment with our mission as a division of the American Chemical Society. | |
Explanation | The data from CAS Common Chemistry is provided under a CC-BY-NC 4.0 license, unless otherwise stated. | |
Record name | L-Glutaminyl-L-glutamic acid | |
Source | EPA DSSTox | |
URL | https://comptox.epa.gov/dashboard/DTXSID501309175 | |
Description | DSSTox provides a high quality public chemistry resource for supporting improved predictive toxicology. | |
Record name | Glutaminylglutamic acid | |
Source | Human Metabolome Database (HMDB) | |
URL | http://www.hmdb.ca/metabolites/HMDB0028796 | |
Description | The Human Metabolome Database (HMDB) is a freely available electronic database containing detailed information about small molecule metabolites found in the human body. | |
Explanation | HMDB is offered to the public as a freely available resource. Use and re-distribution of the data, in whole or in part, for commercial purposes requires explicit permission of the authors and explicit acknowledgment of the source material (HMDB) and the original publication (see the HMDB citing page). We ask that users who download significant portions of the database cite the HMDB paper in any resulting publications. | |
Retrosynthesis Analysis
AI-Powered Synthesis Planning: Our tool employs the Template_relevance Pistachio, Template_relevance Bkms_metabolic, Template_relevance Pistachio_ringbreaker, Template_relevance Reaxys, Template_relevance Reaxys_biocatalysis model, leveraging a vast database of chemical reactions to predict feasible synthetic routes.
One-Step Synthesis Focus: Specifically designed for one-step synthesis, it provides concise and direct routes for your target compounds, streamlining the synthesis process.
Accurate Predictions: Utilizing the extensive PISTACHIO, BKMS_METABOLIC, PISTACHIO_RINGBREAKER, REAXYS, REAXYS_BIOCATALYSIS database, our tool offers high-accuracy predictions, reflecting the latest in chemical research and data.
Strategy Settings
Precursor scoring | Relevance Heuristic |
---|---|
Min. plausibility | 0.01 |
Model | Template_relevance |
Template Set | Pistachio/Bkms_metabolic/Pistachio_ringbreaker/Reaxys/Reaxys_biocatalysis |
Top-N result to add to graph | 6 |
Feasible Synthetic Routes
Q1: What is the role of the Gln-Glu cycle in energy metabolism?
A: The this compound cycle plays a crucial role in regulating energy metabolism, particularly in conditions like chronic hepatic encephalopathy (HE). Research using a rat model with chronic HE induced by thioacetamide (TAA) revealed significant changes in the levels of α-ketoglutarate (αKG) and α-ketoglutaramate (αKGM), key metabolites within the this compound cycle []. These changes were accompanied by decreased activity of ω-amidase (ωA) and glutamine transaminase (GTK), enzymes involved in this metabolic pathway []. This suggests that the this compound cycle significantly contributes to energy regulation during chronic HE.
Q2: How does thymosin β4 influence biological processes?
A: Thymosin β4, a peptide consisting of 43 amino acid residues, has been identified in various tissues, with high concentrations found in the spleen and peritoneal macrophages []. While its exact role is not fully understood, research indicates that thymosin β4 is synthesized in macrophages and spleen cells, suggesting a potential role in immune responses and cellular functions [].
Q3: What is the significance of the αKG/αKGM ratio in chronic HE?
A: The ratio of αKG to αKGM appears to be a potential biomarker for diagnosing the progression of chronic HE []. In the TAA-induced rat model, a substantial increase in αKG levels was observed in blood plasma and organ tissues, while αKGM levels decreased concurrently []. This suggests that monitoring this ratio could be valuable for assessing the severity of HE.
Q4: What is the structure of thymosin β4?
A: Thymosin β4 is a peptide with the following amino acid sequence: Ac-Ser-Asp-Lys-Pro-Asp-Met-Ala-Glu-Ile-Glu-Lys-Phe-Asp-Lys-Ser-Lys-Leu-Lys-Lys-Thr-Glu-Thr-Gln-Glu-Lys-Asn-Pro-Leu-Pro-Ser-Lys-Glu-Thr-Ile-Glu-Gln-Glu-Lys-Gln-Ala-Gly-Glu-Ser-OH [, , ].
Q5: What are some other peptides identified in the provided research and what are their sequences?
A: Several peptides with biological activities were identified. Some examples include:* DSIP (Delta sleep-inducing peptide): Trp-Ala-Gly-Gly-Asp-Ala-Ser-Gly-Glu-OH [, , ]* Cecropin A: Lys-Trp-Lys-Leu-Phe-Lys-Lys-Ile-Glu-Lys-Val-Gly-Gln-Asn-Ile-Arg-Asp-Gly-Ile-Ile-Lys-Ala-Gly-Pro-Ala-Val-Ala-Val-Val-Gly-Gln-Ala-Thr-Gln-Ile-Ala-Lys [, ]* YG-1 (Yeast ACE inhibitor): Gly-His-Lys-Ile-Ala-Thr-Phe-Gln-Glu-Arg []* YG-2 (Yeast ACE inhibitor): Gly-Lys-Lys-Ile-Ala-Thr-Tyr-Gln-Glu-Arg []* YG-3 (Yeast ACE inhibitor): Pro-Ala-Asn-Leu-Pro-Trp-Gly-Ser-Ser-Asn-Val []
Q6: How do structural modifications of the activated protein C (APC) exosite affect its interaction with Factor VIIIa?
A: Research using surface plasmon resonance (SPR) and FXa generation assays revealed that specific basic residues within the APC exosite, particularly Lys39, Arg67, and Arg74, play crucial roles in binding and inactivating Factor VIIIa []. Mutations of these residues, such as Lys39Ala and Arg67Ala, significantly reduced the binding affinity of APC to Factor VIIIa and impaired its ability to inactivate the clotting factor []. These findings highlight the importance of these basic residues in the interaction between APC and Factor VIIIa.
Q7: What is the role of the amino acid sequence 400-429 in HTLV-1 infection?
A: The amino acid sequence 400-429 of the gp21 envelope protein of HTLV-1 is crucial for viral infection, specifically at the postbinding step []. Synthetic peptides derived from this region, particularly gp21 peptide 400-429, significantly inhibited cell-free HTLV-1 infection []. Mutations within this peptide, especially at positions Asn407, Ser408, and Leu413, -419, -424, and -429, diminished its neutralizing activity []. This suggests that this specific region of gp21 is involved in a critical stage of HTLV-1 entry into host cells after initial binding.
Q8: How does the structure of the MDC1 BRCT repeat domain contribute to its function?
A: The tandem BRCT repeats of MDC1, a protein involved in DNA damage response, specifically recognize and bind to the phosphorylated tail of histone variant H2AX (γ-H2AX) []. X-ray crystallography of the MDC1 BRCT domain revealed that this interaction is highly dependent on the presence of a free carboxylate group at the COOH-terminal tyrosine residue of the γ-H2AX tail []. Residues Arg1932 and Arg1933 within the MDC1 BRCT domain are thought to interact with the COOH terminus and penultimate Glu of γ-H2AX, while Gln2013 may contribute to the specific recognition of the COOH-terminal Tyr []. This specific binding is essential for MDC1's role in DNA repair processes.
Q9: How does the this compound-Pro-Val-Leu peptide (QEPVL) impact the immune system?
A: QEPVL, a casein-derived peptide, significantly modulates the immune response by activating lymphocytes []. In vitro and in vivo studies have demonstrated that QEPVL enhances lymphocyte proliferation and increases cyclic AMP levels in a dose-dependent manner []. Furthermore, QEPVL exhibits anti-inflammatory effects by regulating nitric oxide release and modulating cytokine production, such as IL-4, IL-10, IFN-γ, and TNF-α, in vivo []. These findings suggest QEPVL's potential as a functional food ingredient for immune support and inflammation management.
Q10: How does the formulation of thymosin β4 and DSIP as a combination therapy influence its therapeutic potential?
A: Combining thymosin β4 and DSIP as a single therapeutic agent shows promise in addressing various diseases [, , ]. Formulating these peptides together aims to enhance their individual therapeutic effects, potentially leading to improved treatment outcomes for conditions such as cancer, cardiovascular diseases, infections, fibrosis, inflammatory diseases, neurodegenerative diseases, and autoimmune diseases [, , ].
Q11: What are the advantages of lyophilized or liquid buffer formulations for thymosin β4 and DSIP?
A: Lyophilized and liquid buffer formulations offer practical advantages for the administration of thymosin β4 and DSIP, especially for specific patient populations [, ]. These formulations allow for flexible dosing and easier administration, particularly in newborns, toddlers, and preschool children, making them suitable options for delivering these peptides [, ].
Q12: What are the pharmacokinetic properties of NC100668, a potential tracer for venous thromboembolism imaging?
A: NC100668, a novel tracer for imaging venous thromboembolism, exhibits rapid clearance from the bloodstream, with more than half of the injected radioactivity eliminated through urine within 2 hours []. Its pharmacokinetic profile reveals a two-phase elimination process, with an initial rapid half-life of 12 minutes followed by a slower elimination phase with a half-life of 1.2 hours and a terminal half-life of 10.5 hours []. These properties make NC100668 a promising candidate for imaging venous thromboembolism.
Descargo de responsabilidad e información sobre productos de investigación in vitro
Tenga en cuenta que todos los artículos e información de productos presentados en BenchChem están destinados únicamente con fines informativos. Los productos disponibles para la compra en BenchChem están diseñados específicamente para estudios in vitro, que se realizan fuera de organismos vivos. Los estudios in vitro, derivados del término latino "in vidrio", involucran experimentos realizados en entornos de laboratorio controlados utilizando células o tejidos. Es importante tener en cuenta que estos productos no se clasifican como medicamentos y no han recibido la aprobación de la FDA para la prevención, tratamiento o cura de ninguna condición médica, dolencia o enfermedad. Debemos enfatizar que cualquier forma de introducción corporal de estos productos en humanos o animales está estrictamente prohibida por ley. Es esencial adherirse a estas pautas para garantizar el cumplimiento de los estándares legales y éticos en la investigación y experimentación.