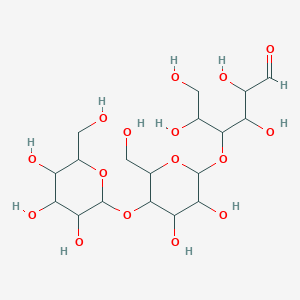
maltotriosa
- Haga clic en CONSULTA RÁPIDA para recibir una cotización de nuestro equipo de expertos.
- Con productos de calidad a un precio COMPETITIVO, puede centrarse más en su investigación.
Descripción general
Descripción
Aplicaciones Científicas De Investigación
maltotriose has several applications in scientific research and industry. It is used in the food industry for its mild sweetness and moisture-regulating properties . In the pharmaceutical industry, maltotriose and its derivatives are used in nutritional products and functional foods due to their prebiotic effects . maltotriose also plays a role in fermentation processes, particularly in the production of ethanol and other fermented products .
Mecanismo De Acción
Target of Action
Maltotriose, a trisaccharide consisting of three glucose molecules linked with α-1,4 glycosidic bonds , primarily targets enzymes such as α-amylase and maltotriose-forming amylase (EC 3.2.1.133) . These enzymes play a crucial role in carbohydrate metabolism, particularly in the hydrolysis of starch .
Mode of Action
Maltotriose interacts with its target enzymes through a process known as enzymatic hydrolysis . In this process, the enzymes break down the α-1,4 glycosidic bonds in maltotriose, converting it into glucose units . This enzymatic action is facilitated by the active sites on the enzymes, which bind to the maltotriose molecule and catalyze the hydrolysis reaction .
Biochemical Pathways
The primary biochemical pathway affected by maltotriose is the starch metabolism pathway . In this pathway, maltotriose is actively transported into the cell and hydrolyzed by intracellular α-glucosidases . The resulting glucose units can then enter glycolysis, a metabolic pathway that converts glucose into pyruvate, releasing energy in the form of ATP .
Pharmacokinetics
It is known that maltotriose is most commonly produced by the digestive enzyme alpha-amylase on amylose in starch , suggesting that it is likely absorbed in the digestive tract and metabolized into glucose units .
Result of Action
The enzymatic hydrolysis of maltotriose results in the production of glucose units . These glucose units can be used by cells for energy production through the process of glycolysis . In certain strains of yeast, such as Saccharomyces cerevisiae, the fermentation of maltotriose can also lead to the production of ethanol .
Action Environment
The action of maltotriose is influenced by various environmental factors. For instance, the activity of the enzymes that hydrolyze maltotriose can be affected by factors such as temperature and pH . Additionally, the presence of other sugars, such as sucrose, can influence the metabolism of maltotriose in yeast cells .
Análisis Bioquímico
Biochemical Properties
Maltotriose interacts with several enzymes and proteins within cells. In the yeast Saccharomyces cerevisiae, maltotriose is actively transported into the cell by the AGT1 permease, a transporter required for maltotriose utilization . Once inside the cell, maltotriose is hydrolyzed by intracellular α-glucosidases .
Cellular Effects
Maltotriose influences various cellular processes. In yeast cells, maltotriose is metabolized through a process that involves its active transport into the cell and subsequent hydrolysis . This process impacts cellular metabolism, contributing to the energy production within the cell .
Molecular Mechanism
Maltotriose exerts its effects at the molecular level through its interactions with specific biomolecules. It is transported into the cell by the AGT1 permease and is then hydrolyzed by intracellular α-glucosidases . This process allows maltotriose to be broken down into simpler sugars that can be used in cellular metabolism .
Temporal Effects in Laboratory Settings
In laboratory settings, the effects of maltotriose can change over time. For instance, in yeast fermentation processes, the efficiency of maltotriose fermentation can be influenced by the expression levels of the AGT1 permease and intracellular α-glucosidases .
Metabolic Pathways
Maltotriose is involved in several metabolic pathways. In yeast cells, maltotriose is metabolized through a pathway that involves its active transport into the cell by the AGT1 permease and subsequent hydrolysis by intracellular α-glucosidases .
Transport and Distribution
Maltotriose is transported into yeast cells by the AGT1 permease . Once inside the cell, it is distributed and metabolized, contributing to various cellular processes .
Subcellular Localization
Within yeast cells, maltotriose is localized in the cytoplasm following its transport into the cell by the AGT1 permease . It is then hydrolyzed by intracellular α-glucosidases, allowing it to contribute to cellular metabolism .
Métodos De Preparación
Synthetic Routes and Reaction Conditions: maltotriose can be synthesized by hydrolyzing pullulan with pullulanase. The optimal conditions for this reaction are a pH of 5.0, a temperature of 45°C, and a reaction time of 6 hours . The hydrolysate is then filtered, concentrated, and precipitated with ethanol to obtain maltotriose .
Industrial Production Methods: Industrial production of maltotriose often involves the use of maltotriose-producing amylases derived from microorganisms such as Streptomyces, Bacillus, and Microbacterium . These amylases hydrolyze starch to produce maltotriose as the primary product .
Análisis De Reacciones Químicas
Types of Reactions: maltotriose primarily undergoes hydrolysis reactions. It can be hydrolyzed by alpha-amylase to produce glucose and maltose .
Common Reagents and Conditions: The hydrolysis of maltotriose typically requires enzymes such as alpha-amylase and specific conditions like a pH range of 6.0 to 6.5 and a temperature range of 44.95°C to 50°C .
Major Products Formed: The major products formed from the hydrolysis of maltotriose are glucose and maltose .
Comparación Con Compuestos Similares
maltotriose is similar to other glucose-based oligosaccharides such as maltose and maltotetraose . it is unique in its structure, consisting of three glucose molecules linked by α-1,4 glycosidic bonds . This structure gives maltotriose distinct properties, such as its mild sweetness and ability to regulate moisture . Other similar compounds include acarbose, a pseudo-saccharide that also elicits a sweet taste .
Propiedades
IUPAC Name |
4-[3,4-dihydroxy-6-(hydroxymethyl)-5-[3,4,5-trihydroxy-6-(hydroxymethyl)oxan-2-yl]oxyoxan-2-yl]oxy-2,3,5,6-tetrahydroxyhexanal |
Source
|
---|---|---|
Details | Computed by Lexichem TK 2.7.0 (PubChem release 2021.05.07) | |
Source | PubChem | |
URL | https://pubchem.ncbi.nlm.nih.gov | |
Description | Data deposited in or computed by PubChem | |
InChI |
InChI=1S/C18H32O16/c19-1-5(23)9(25)15(6(24)2-20)33-18-14(30)12(28)16(8(4-22)32-18)34-17-13(29)11(27)10(26)7(3-21)31-17/h1,5-18,20-30H,2-4H2 |
Source
|
Details | Computed by InChI 1.0.6 (PubChem release 2021.05.07) | |
Source | PubChem | |
URL | https://pubchem.ncbi.nlm.nih.gov | |
Description | Data deposited in or computed by PubChem | |
InChI Key |
RXVWSYJTUUKTEA-UHFFFAOYSA-N |
Source
|
Details | Computed by InChI 1.0.6 (PubChem release 2021.05.07) | |
Source | PubChem | |
URL | https://pubchem.ncbi.nlm.nih.gov | |
Description | Data deposited in or computed by PubChem | |
Canonical SMILES |
C(C1C(C(C(C(O1)OC2C(OC(C(C2O)O)OC(C(CO)O)C(C(C=O)O)O)CO)O)O)O)O |
Source
|
Details | Computed by OEChem 2.3.0 (PubChem release 2021.05.07) | |
Source | PubChem | |
URL | https://pubchem.ncbi.nlm.nih.gov | |
Description | Data deposited in or computed by PubChem | |
Molecular Formula |
C18H32O16 |
Source
|
Details | Computed by PubChem 2.1 (PubChem release 2021.05.07) | |
Source | PubChem | |
URL | https://pubchem.ncbi.nlm.nih.gov | |
Description | Data deposited in or computed by PubChem | |
Molecular Weight |
504.4 g/mol |
Source
|
Details | Computed by PubChem 2.1 (PubChem release 2021.05.07) | |
Source | PubChem | |
URL | https://pubchem.ncbi.nlm.nih.gov | |
Description | Data deposited in or computed by PubChem | |
CAS No. |
1109-28-0 |
Source
|
Record name | maltotriose | |
Source | DTP/NCI | |
URL | https://dtp.cancer.gov/dtpstandard/servlet/dwindex?searchtype=NSC&outputformat=html&searchlist=170180 | |
Description | The NCI Development Therapeutics Program (DTP) provides services and resources to the academic and private-sector research communities worldwide to facilitate the discovery and development of new cancer therapeutic agents. | |
Explanation | Unless otherwise indicated, all text within NCI products is free of copyright and may be reused without our permission. Credit the National Cancer Institute as the source. | |
Descargo de responsabilidad e información sobre productos de investigación in vitro
Tenga en cuenta que todos los artículos e información de productos presentados en BenchChem están destinados únicamente con fines informativos. Los productos disponibles para la compra en BenchChem están diseñados específicamente para estudios in vitro, que se realizan fuera de organismos vivos. Los estudios in vitro, derivados del término latino "in vidrio", involucran experimentos realizados en entornos de laboratorio controlados utilizando células o tejidos. Es importante tener en cuenta que estos productos no se clasifican como medicamentos y no han recibido la aprobación de la FDA para la prevención, tratamiento o cura de ninguna condición médica, dolencia o enfermedad. Debemos enfatizar que cualquier forma de introducción corporal de estos productos en humanos o animales está estrictamente prohibida por ley. Es esencial adherirse a estas pautas para garantizar el cumplimiento de los estándares legales y éticos en la investigación y experimentación.