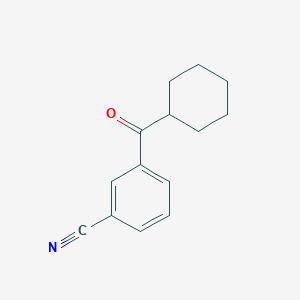
3-Cyanophenyl cyclohexyl ketone
- Haga clic en CONSULTA RÁPIDA para recibir una cotización de nuestro equipo de expertos.
- Con productos de calidad a un precio COMPETITIVO, puede centrarse más en su investigación.
Descripción general
Descripción
Métodos De Preparación
Synthetic Routes and Reaction Conditions
One of the common methods for synthesizing 3-Cyanophenyl cyclohexyl ketone involves the Suzuki–Miyaura coupling reaction. This reaction is widely applied for forming carbon–carbon bonds under mild and functional group tolerant conditions . The process typically involves the use of organoboron reagents and palladium catalysts .
Industrial Production Methods
Industrial production methods for cyclohexyl phenyl ketones, including this compound, often involve the hydrogenation of carbon-to-carbon double or triple bonds . This method is efficient and does not require purification or separation steps .
Análisis De Reacciones Químicas
Types of Reactions
3-Cyanophenyl cyclohexyl ketone undergoes various types of chemical reactions, including:
Oxidation: The compound can be oxidized to form corresponding carboxylic acids.
Reduction: Reduction reactions can convert the ketone group to an alcohol.
Substitution: The compound can undergo substitution reactions, particularly at the cyano group.
Common Reagents and Conditions
Oxidation: Common oxidizing agents include potassium permanganate (KMnO4) and chromium trioxide (CrO3).
Reduction: Reducing agents such as lithium aluminum hydride (LiAlH4) and sodium borohydride (NaBH4) are commonly used.
Substitution: Reagents like sodium methoxide (NaOCH3) and potassium tert-butoxide (KOtBu) are often employed.
Major Products Formed
Oxidation: Carboxylic acids.
Reduction: Alcohols.
Substitution: Various substituted derivatives depending on the reagent used.
Aplicaciones Científicas De Investigación
3-Cyanophenyl cyclohexyl ketone has a wide range of applications in scientific research:
Chemistry: Used as an intermediate in organic synthesis and in the preparation of heterocyclic compounds.
Biology: Employed in the study of enzyme inhibition and protein-ligand interactions.
Medicine: Investigated for its potential therapeutic properties, including anti-inflammatory and analgesic effects.
Industry: Utilized in the production of pharmaceuticals, agrochemicals, and specialty chemicals.
Mecanismo De Acción
The mechanism of action of 3-Cyanophenyl cyclohexyl ketone involves its interaction with specific molecular targets and pathways. The compound can act as an inhibitor of certain enzymes, affecting their activity and leading to various biological effects . The exact molecular targets and pathways depend on the specific application and context of use.
Comparación Con Compuestos Similares
Similar Compounds
Cyclohexyl phenyl ketone: Similar in structure but lacks the cyano group.
Benzonitrile derivatives: Compounds with similar cyano groups but different substituents on the aromatic ring.
Uniqueness
3-Cyanophenyl cyclohexyl ketone is unique due to the presence of both the cyclohexyl ketone and cyano functional groups. This combination imparts distinct chemical and biological properties, making it valuable for various research applications .
Actividad Biológica
3-Cyanophenyl cyclohexyl ketone (C₁₄H₁₅NO) is a chemical compound with notable biological activity, particularly in the fields of medicinal chemistry and pharmacology. This article explores its biological properties, mechanisms of action, and potential therapeutic applications, supported by data tables and relevant research findings.
Chemical Structure and Properties
This compound features a cyano group attached to a phenyl ring at the meta position, along with a cyclohexyl ketone structure. This unique arrangement contributes to its reactivity and biological interactions.
Property | Value |
---|---|
Molecular Formula | C₁₄H₁₅NO |
Molecular Weight | 225.28 g/mol |
Appearance | White to light yellow solid |
Solubility | Soluble in organic solvents |
The biological activity of this compound is primarily attributed to its ability to interact with specific enzymes and receptors. It has been identified as an enzyme inhibitor , which can modulate various biochemical pathways. The following mechanisms have been proposed:
- Enzyme Inhibition : The compound may inhibit enzymes involved in inflammatory pathways, leading to potential anti-inflammatory effects.
- Protein-Ligand Interactions : It can bind to proteins, influencing their activity and stability, which is crucial for therapeutic applications.
Anti-inflammatory Effects
Research indicates that this compound exhibits significant anti-inflammatory properties. A study demonstrated its efficacy in reducing inflammation markers in vitro, suggesting potential applications in treating inflammatory diseases.
Analgesic Properties
In addition to anti-inflammatory effects, this compound has been explored for its analgesic properties. It has shown promise in pain relief models, indicating its potential as a non-opioid pain management option.
Case Studies and Research Findings
- Inhibition of Enzymes : A study published in Nature reported that compounds similar to this compound demonstrated inhibition of carbonic anhydrase II (CA-II), which is relevant for developing treatments for conditions like glaucoma and epilepsy .
- Pharmacological Screening : In a pharmacological screening of various derivatives, this compound was assessed for its binding affinity to several receptors. The results indicated moderate affinity towards certain G-protein coupled receptors (GPCRs), highlighting its potential as a lead compound for drug development.
- Analgesic Activity Testing : In animal models, the compound was tested for analgesic effects using the hot plate test. Results showed a significant reduction in pain response times compared to control groups, suggesting effective analgesic properties.
Applications in Medicinal Chemistry
The compound's unique structure allows it to serve as a valuable intermediate in organic synthesis, particularly in the development of new pharmaceuticals. Its potential applications include:
- Drug Development : As a scaffold for designing new anti-inflammatory and analgesic drugs.
- Research Tool : Used in studies investigating enzyme inhibition and receptor interactions.
Propiedades
IUPAC Name |
3-(cyclohexanecarbonyl)benzonitrile |
Source
|
---|---|---|
Source | PubChem | |
URL | https://pubchem.ncbi.nlm.nih.gov | |
Description | Data deposited in or computed by PubChem | |
InChI |
InChI=1S/C14H15NO/c15-10-11-5-4-8-13(9-11)14(16)12-6-2-1-3-7-12/h4-5,8-9,12H,1-3,6-7H2 |
Source
|
Source | PubChem | |
URL | https://pubchem.ncbi.nlm.nih.gov | |
Description | Data deposited in or computed by PubChem | |
InChI Key |
ZBSJNGQDXHAFOF-UHFFFAOYSA-N |
Source
|
Source | PubChem | |
URL | https://pubchem.ncbi.nlm.nih.gov | |
Description | Data deposited in or computed by PubChem | |
Canonical SMILES |
C1CCC(CC1)C(=O)C2=CC=CC(=C2)C#N |
Source
|
Source | PubChem | |
URL | https://pubchem.ncbi.nlm.nih.gov | |
Description | Data deposited in or computed by PubChem | |
Molecular Formula |
C14H15NO |
Source
|
Source | PubChem | |
URL | https://pubchem.ncbi.nlm.nih.gov | |
Description | Data deposited in or computed by PubChem | |
DSSTOX Substance ID |
DTXSID20642587 |
Source
|
Record name | 3-(Cyclohexanecarbonyl)benzonitrile | |
Source | EPA DSSTox | |
URL | https://comptox.epa.gov/dashboard/DTXSID20642587 | |
Description | DSSTox provides a high quality public chemistry resource for supporting improved predictive toxicology. | |
Molecular Weight |
213.27 g/mol |
Source
|
Source | PubChem | |
URL | https://pubchem.ncbi.nlm.nih.gov | |
Description | Data deposited in or computed by PubChem | |
CAS No. |
898792-11-5 |
Source
|
Record name | 3-(Cyclohexanecarbonyl)benzonitrile | |
Source | EPA DSSTox | |
URL | https://comptox.epa.gov/dashboard/DTXSID20642587 | |
Description | DSSTox provides a high quality public chemistry resource for supporting improved predictive toxicology. | |
Descargo de responsabilidad e información sobre productos de investigación in vitro
Tenga en cuenta que todos los artículos e información de productos presentados en BenchChem están destinados únicamente con fines informativos. Los productos disponibles para la compra en BenchChem están diseñados específicamente para estudios in vitro, que se realizan fuera de organismos vivos. Los estudios in vitro, derivados del término latino "in vidrio", involucran experimentos realizados en entornos de laboratorio controlados utilizando células o tejidos. Es importante tener en cuenta que estos productos no se clasifican como medicamentos y no han recibido la aprobación de la FDA para la prevención, tratamiento o cura de ninguna condición médica, dolencia o enfermedad. Debemos enfatizar que cualquier forma de introducción corporal de estos productos en humanos o animales está estrictamente prohibida por ley. Es esencial adherirse a estas pautas para garantizar el cumplimiento de los estándares legales y éticos en la investigación y experimentación.