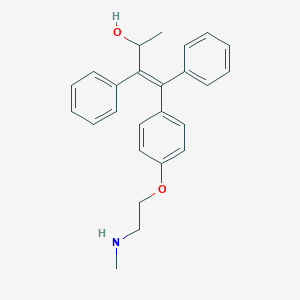
alpha-Hydroxy-N-desmethyltamoxifen
Descripción general
Descripción
Alpha-hydroxy-N-desmethyltamoxifen (α-OH-NDT) is a secondary metabolite of tamoxifen, a nonsteroidal antiestrogen widely used in breast cancer treatment and prevention . This metabolite is generated through sequential metabolic steps: tamoxifen is first N-demethylated to form N-desmethyltamoxifen (NDT), which is subsequently α-hydroxylated to produce α-OH-NDT . Unlike its parent compound, α-OH-NDT is implicated in genotoxic pathways due to its ability to form DNA adducts. Specifically, esterification (e.g., sulfation) of α-OH-NDT generates a reactive carbocation that binds covalently to DNA, predominantly forming (E)-α-(deoxyguanosin-N²-yl)-N-desmethyltamoxifen adducts in vitro and in vivo . The R-isomer of α-OH-NDT is 10-fold more potent in adduct formation than the S-isomer in rat hepatocytes, attributed to its superior substrate specificity for sulfotransferases .
Métodos De Preparación
Synthetic Routes to α-Hydroxy-N-desmethyltamoxifen
Derivatization and Diastereomeric Separation
The most detailed preparation method involves the synthesis of α-OH-NDTAM enantiomers through derivatization with a chiral auxiliary. Starting with N-desmethyltamoxifen, the α-hydroxyl group is protected as an S-camphanate ester. The protocol, as described by Osborne et al., proceeds as follows :
-
Esterification : N-Desmethyltamoxifen is treated with S-camphanic acid chloride in the presence of a base to form N-ethoxycarbonyl-N-desmethyltamoxifen-α-S-camphanate.
-
Diastereomer Separation : The resulting diastereoisomers are separated using silica gel chromatography, exploiting differences in polarity.
-
Hydrolysis : Each diastereoisomer is hydrolyzed under mild acidic conditions (e.g., dilute HCl in methanol) to yield the corresponding (+)- and (−)-α-OH-NDTAM enantiomers.
This method ensures high enantiomeric purity, critical for studying stereospecific biological effects. The (−)-enantiomer was confirmed as the S-configuration through degradation to (−)-2-hydroxy-1-phenyl-1-propanone, a compound with established stereochemistry .
Chiral Resolution and Stereochemical Analysis
Determination of Absolute Configuration
The absolute configuration of α-OH-NDTAM enantiomers was resolved through chemical degradation and comparative analysis. Hydrolysis of the (−)-enantiomer’s camphanate ester produced a fragment identical to (−)-2-hydroxy-1-phenyl-1-propanone, which has a known S-configuration . This confirmed the (−)-enantiomer as S-α-OH-NDTAM and the (+)-enantiomer as R-α-OH-NDTAM.
Impact of Stereochemistry on DNA Adduct Formation
Enantiomeric differences significantly influence genotoxicity. In rat hepatocytes, R-α-OH-NDTAM formed 10 times more DNA adducts than the S-enantiomer . This disparity highlights the necessity of stereochemical control in pharmacokinetic and toxicological studies.
Analytical Characterization Techniques
Nuclear Magnetic Resonance (NMR) Spectroscopy
Proton NMR spectroscopy has been pivotal in characterizing α-OH-NDTAM and its derivatives. Key spectral features include:
-
Aromatic protons : Resonances between δ 6.5–7.5 ppm, indicative of the triphenylethylene backbone.
-
Methylene groups : Signals near δ 2.8–3.2 ppm, corresponding to the N-desmethyl moiety.
-
Hydroxyl proton : A broad singlet at δ 5.2 ppm, absent in esterified derivatives .
Mass Spectrometry (MS)
High-resolution MS (HRMS) confirms molecular integrity. For α-OH-NDTAM ([C<sub>25</sub>H<sub>29</sub>NO<sub>2</sub>]<sup>+</sup>), the observed m/z of 382.2147 aligns with the theoretical value (Δ < 2 ppm) . Fragmentation patterns further validate the hydroxyl group’s position.
Applications in Genotoxicity Research
DNA Adduct Profiling
α-OH-NDTAM’s reactivity with DNA has been studied using <sup>32</sup>P-postlabeling and LC-MS/MS. Major adducts include:
-
α-(N<sup>2</sup>-Deoxyguanosinyl)-N-desmethyltamoxifen (dG-N<sup>2</sup>-NDTAM) : Formed via reaction of α-acetoxy-NDTAM with 2′-deoxyguanosine .
-
Trans vs. Cis Isomers : Adduct stereochemistry affects repair kinetics and mutagenic potential .
Comparative Species Metabolism
Rodent vs. human metabolic studies reveal species-specific differences. Rat hepatocytes generate 10-fold more DNA adducts from R-α-OH-NDTAM than human hepatocytes, suggesting divergent detoxification pathways .
Reaction Optimization and Yield Considerations
Step | Reagents/Conditions | Yield/Outcome |
---|---|---|
Esterification | S-Camphanic acid chloride, base | Quantitative conversion |
Diastereomer Separation | Silica gel chromatography | >95% purity per diastereoisomer |
Hydrolysis | 0.1 M HCl in methanol, 25°C, 2 h | 85–90% recovery |
Análisis De Reacciones Químicas
Sulfation and DNA Adduct Formation
α-OH-NDMTAM undergoes esterification via sulfotransferases to form a highly reactive α-sulfoxy intermediate. This electrophilic species reacts with DNA bases, predominantly forming covalent adducts at the N²-position of deoxyguanosine:
-
Primary adduct : (E)-α-(deoxyguanosin-N²-yl)-N-desmethyltamoxifen is the major DNA adduct detected in rat liver cells treated with α-OH-NDMTAM .
-
Adduct specificity : The adduct profile matches that observed in vivo when rats are administered tamoxifen or α-hydroxytamoxifen, confirming its role in tamoxifen-induced genotoxicity .
Table 1: DNA Adduct Levels in Rat Liver After Metabolite Exposure
Metabolite | Relative Adduct Level (RAL) | Key Adduct Identified |
---|---|---|
α-OH-NDMTAM | 1.0 (reference) | (E)-α-(deoxyguanosin-N²-yl) |
Tamoxifen | 0.8–1.2 | Mixed adducts |
N,N-Didesmethyltamoxifen | 0.3–0.5 | Minor adducts |
Data derived from in vivo rat studies .
Enantiomer-Specific Reactivity
The stereochemistry of α-OH-NDMTAM significantly influences its metabolic activation:
-
R-(+)-enantiomer : Generates 8–10× higher DNA adduct levels in rat hepatocytes compared to the S-(−)-enantiomer due to enhanced sulfotransferase substrate affinity .
-
S-(−)-enantiomer : Exhibits reduced reactivity, contributing minimally to adduct formation despite similar chemical stability .
Phase II Conjugation Pathways
While sulfation dominates, α-OH-NDMTAM also participates in:
-
Glucuronidation : Detected as a minor pathway in human hepatic microsomes, forming inert glucuronides for excretion .
-
N-Dealkylation : Further metabolized by CYP3A4/5 to N,N-didesmethyltamoxifen, which undergoes additional activation to form secondary adducts .
Comparative Activation with Related Metabolites
α-OH-NDMTAM exhibits intermediate reactivity compared to other tamoxifen metabolites:
-
α-Hydroxytamoxifen : Higher adduct formation (RAL = 1.5–2.0) .
-
4-Hydroxytamoxifen : Minimal DNA binding, primarily associated with estrogen receptor antagonism .
In Vitro vs. In Vivo Reactivity
-
In vitro : α-Sulfoxy-NDMTAM reacts directly with DNA to form adducts, confirmed by ³²P-postlabeling and HPLC .
-
In vivo : Adduct levels correlate with hepatocarcinogenicity in rats but are absent in non-hepatic tissues (e.g., uterus, spleen) .
Role in Human Toxicity
Despite its genotoxicity in rats, α-OH-NDMTAM’s contribution to human endometrial cancer remains unclear due to:
Aplicaciones Científicas De Investigación
Pharmacological Characterization
Mechanism of Action
Alpha-Hydroxy-N-desmethyltamoxifen exerts its effects primarily through binding to estrogen receptors. This interaction inhibits the proliferation of estrogen-dependent breast cancer cells, contributing to its anticancer properties. The compound is metabolically activated by cytochrome P450 enzymes, particularly CYP2D6, which converts it into endoxifen, another active form with high affinity for estrogen receptors .
Key Findings
- Binding Affinity : Studies indicate that this compound has a significant binding affinity for estrogen receptors, which is essential for its role in inhibiting cancer cell growth .
- Antitumor Activity : In vivo studies have shown that this compound can significantly inhibit tumor growth in xenograft models, with inhibition rates reaching up to 60% at doses of 20 mg/kg.
Scientific Research Applications
Field | Application |
---|---|
Chemistry | Used as a reference compound in metabolic studies involving tamoxifen derivatives. |
Biology | Investigated for its effects on cellular processes related to estrogen modulation. |
Medicine | Critical in understanding the pharmacokinetics and pharmacodynamics of tamoxifen therapy. |
Industry | Aids in the development of new selective estrogen receptor modulators (SERMs). |
Case Study 1: Anticancer Effects
- Objective : Evaluate the anticancer effects in breast cancer models.
- Results : The study demonstrated significant apoptosis induction in cancer cells treated with this compound while showing minimal effects on normal cells.
Case Study 2: Inflammation Reduction
- Objective : Assess anti-inflammatory efficacy in models of induced arthritis.
- Results : Significant reductions in paw swelling were observed after treatment with the compound, indicating potential therapeutic benefits beyond oncology.
Case Study 3: Antimicrobial Activity
- Objective : Investigate antimicrobial efficacy against resistant bacterial strains.
- Results : this compound showed effective inhibition of growth in multi-drug resistant bacterial strains, suggesting a broader application in infection control.
In Vivo Studies
In vivo studies have highlighted the efficacy of this compound across various animal models:
- Antitumor Activity : Demonstrated significant tumor growth inhibition compared to control groups.
- Anti-inflammatory Effects : Showed promise in reducing inflammation markers, which could be beneficial for conditions like arthritis.
Mecanismo De Acción
The mechanism of action of alpha-Hydroxy-N-desmethyltamoxifen involves its interaction with estrogen receptors. It binds to these receptors, inhibiting the proliferation of estrogen-dependent breast cancer cells. The compound is metabolically activated by CYP2D6, which converts it into its active form, endoxifen. This active form has a high affinity for estrogen receptors and effectively inhibits their activity, leading to reduced cancer cell growth .
Comparación Con Compuestos Similares
Tamoxifen
- Structure: C₂₆H₂₉NO (base structure with dimethylaminoethoxy and phenyl groups).
- Role: Primary antiestrogen drug; undergoes extensive hepatic metabolism to active and genotoxic metabolites.
- DNA Adduct Formation : Indirect via α-hydroxylation to α-hydroxytamoxifen (α-OH-TAM) and α-OH-NDT. Adduct levels in rat liver: α-OH-TAM > tamoxifen ≈ NDT ≈ α-OH-NDT .
- Clinical Impact : Reduces breast cancer risk by 49% but increases endometrial cancer risk (2.5-fold) .
N-Desmethyltamoxifen (NDT)
- Structure: C₂₅H₂₇NO (demethylated analog of tamoxifen).
- Role : Major circulating metabolite with longer half-life than tamoxifen; precursor to α-OH-NDT and 4-hydroxy-N-desmethyltamoxifen (endoxifen).
- DNA Adduct Formation: Requires α-hydroxylation to α-OH-NDT for genotoxicity. Adduct levels in rat liver are comparable to tamoxifen .
4-Hydroxy-N-desmethyltamoxifen (Endoxifen)
- Structure: C₂₅H₂₇NO₂ (hydroxylated at the 4-position of the phenyl group).
- Role : Most potent antiestrogenic metabolite (100-fold higher estrogen receptor affinity than tamoxifen) .
- Clinical Impact: Primary mediator of tamoxifen’s therapeutic effects; minimal role in carcinogenicity.
Alpha-Hydroxytamoxifen (α-OH-TAM)
- Structure: C₂₆H₂₉NO₂ (α-hydroxylated tamoxifen).
- Role: Direct genotoxic metabolite; forms DNA adducts without requiring further metabolism.
- DNA Adduct Formation : Highest adduct levels among tamoxifen metabolites in rat liver (R-isomer forms 8× more adducts than S-isomer) .
- Clinical Impact: Key contributor to tamoxifen’s hepatocarcinogenicity in rats; human relevance under investigation.
Comparative Data Table
Key Research Findings
Stereoselectivity in Genotoxicity: The R-isomers of α-OH-NDT and α-OH-TAM are significantly more genotoxic than their S-counterparts due to enhanced sulfotransferase-mediated activation .
Human Relevance : While α-OH-NDT adducts are observed in human endometrial tissue, their contribution to endometrial cancer remains unclear .
Therapeutic vs. Toxic Metabolites: Endoxifen (non-genotoxic) and α-OH-NDT (genotoxic) exemplify the dual role of tamoxifen metabolism in efficacy and toxicity .
Actividad Biológica
Alpha-Hydroxy-N-desmethyltamoxifen (α-OH-N-DMT) is a metabolite of tamoxifen, a widely used antiestrogen in the treatment of hormone receptor-positive breast cancer. Understanding the biological activity of α-OH-N-DMT is crucial, as it plays a significant role in the drug's efficacy and safety profile. This article explores the biological activity of α-OH-N-DMT, focusing on its mechanisms of action, genotoxicity, and implications for cancer treatment.
Tamoxifen is metabolized into several active forms, including α-OH-N-DMT. This compound is primarily formed through the metabolic pathway involving cytochrome P450 enzymes, particularly CYP2D6. The biological activity of α-OH-N-DMT is attributed to its ability to bind to estrogen receptors and its potential to form DNA adducts, which can lead to genotoxic effects.
Estrogen Receptor Binding
Research indicates that α-OH-N-DMT exhibits antiestrogenic properties similar to tamoxifen. It binds selectively to estrogen receptors (ERα and ERβ), influencing gene expression related to cell growth and differentiation. This binding can inhibit estrogen-mediated proliferation in breast cancer cells, contributing to the therapeutic effects observed with tamoxifen treatment .
Genotoxicity and DNA Adduct Formation
One of the critical aspects of α-OH-N-DMT's biological activity is its potential genotoxicity. Studies have demonstrated that this compound can form DNA adducts through metabolic activation, leading to mutations and potentially contributing to carcinogenesis.
DNA Adducts
The formation of DNA adducts occurs when α-OH-N-DMT is activated through sulfation or other metabolic processes. The major adduct identified is (E)-alpha-(deoxyguanosin-N(2)-yl)-N-desmethyltamoxifen, which has been characterized using high-performance liquid chromatography (HPLC) and post-labeling techniques .
Table 1: Major DNA Adducts Formed by α-OH-N-DMT
Adduct Name | Formation Mechanism | Source |
---|---|---|
(E)-alpha-(deoxyguanosin-N(2)-yl)-N-DMT | Sulfation followed by hydrolysis | Rat liver studies |
N-desmethyltamoxifen adduct | Direct reaction with DNA | In vitro studies |
Case Studies and Clinical Implications
Several studies have examined the clinical implications of α-OH-N-DMT in breast cancer therapy. The variable metabolism of tamoxifen among patients due to genetic polymorphisms in CYP2D6 can lead to differences in the levels of α-OH-N-DMT and other metabolites, impacting treatment outcomes.
Case Study Insights
- CYP2D6 Variants : Patients with different CYP2D6 genotypes exhibit varying plasma concentrations of active metabolites. Those with poor metabolizer phenotypes show significantly lower levels of α-OH-N-DMT, which correlates with reduced therapeutic efficacy .
- Adverse Effects : The formation of DNA adducts by α-OH-N-DMT raises concerns about potential long-term risks associated with tamoxifen therapy, including an increased risk of endometrial cancer and liver tumors observed in animal models .
Q & A
Basic Research Questions
Q. What is the role of alpha-hydroxy-N-desmethyltamoxifen in tamoxifen’s metabolic pathway, and how is its genotoxicity assessed experimentally?
this compound is a secondary metabolite of tamoxifen, formed via cytochrome P450-mediated oxidation. It is genotoxic due to its ability to form covalent DNA adducts, primarily in hepatic tissues. Experimental assessment involves incubating rat hepatocytes with the metabolite, followed by DNA extraction and quantification of adducts using P-postlabeling or LC-MS/MS. Comparative studies between enantiomers (R- and S-isomers) reveal stereoselective adduct formation, with the R-isomer showing 10-fold higher DNA binding .
Q. What synthetic methodologies are used to produce enantiomerically pure this compound for mechanistic studies?
Enantiomeric resolution typically involves derivatization with chiral auxiliaries. For example, N-ethoxycarbonyl-N-desmethyltamoxifen-alpha-S-camphanate is synthesized, separated into diastereoisomers via column chromatography, and hydrolyzed to yield (+)-R and (−)-S enantiomers. Configuration is confirmed through degradation to derivatives with known stereochemistry (e.g., (−)-2-hydroxy-1-phenyl-1-propanone). Purity is validated via chiral HPLC and NMR .
Q. How do researchers detect and quantify DNA adducts formed by this compound in vitro?
Adducts are detected using P-postlabeling coupled with thin-layer chromatography (TLC) or liquid chromatography–tandem mass spectrometry (LC-MS/MS). In vitro protocols involve incubating the metabolite with DNA at physiological pH (e.g., pH 6.0) for 24–48 hours. Adduct levels are normalized to total DNA content, with positive controls (e.g., benzo[a]pyrene-derived adducts) ensuring assay validity .
Advanced Research Questions
Q. Why does the R-isomer of this compound exhibit significantly higher DNA adduct formation in vivo compared to the S-isomer, despite similar in vitro reactivity?
The R-isomer is preferentially sulfonated by hepatic sulfotransferases (SULTs), generating a reactive carbocation that binds DNA. In contrast, the S-isomer has lower affinity for SULTs, reducing its metabolic activation. This stereoselectivity was demonstrated in rat hepatocytes, where R-(+)-alpha-hydroxy-N-desmethyltamoxifen produced 10-fold more adducts than the S-(−)-form. Knockdown of SULT1A1 in vitro models can validate this mechanism .
Q. How can conflicting data on the carcinogenic potential of this compound be reconciled across different experimental models?
Discrepancies arise from variations in metabolic competence (e.g., SULT expression levels in cell lines vs. primary hepatocytes) and DNA repair efficiency. For example, immortalized cell lines may lack functional repair enzymes, amplifying adduct persistence. Standardizing models (e.g., using Big Blue transgenic rats for lacI mutation assays) and controlling for enzyme activity via proteomic profiling can mitigate contradictions .
Q. What experimental designs are optimal for evaluating the in vivo genotoxicity of this compound?
Use transgenic rodent models (e.g., Big Blue rats) to quantify mutations in target genes like lacI. Administer the metabolite via intraperitoneal injection, with dosing regimens reflecting human pharmacokinetics. Terminate studies at 24–72 hours post-exposure to capture acute DNA damage. Include controls for spontaneous mutations and validate adduct formation via LC-MS/MS .
Q. What analytical techniques differentiate this compound adducts from those formed by other tamoxifen metabolites (e.g., alpha-hydroxytamoxifen)?
High-resolution mass spectrometry (HRMS) with collision-induced dissociation (CID) distinguishes adducts based on fragmentation patterns. For example, this compound adducts lack the N-methyl group, yielding characteristic mass shifts (−14.02 Da) compared to alpha-hydroxytamoxifen. Isotopic labeling (e.g., C-tamoxifen) can further track metabolite-specific adducts .
Q. Methodological Guidance
Q. How should researchers design dose-response studies to assess this compound’s genotoxicity?
- In vitro: Use primary hepatocytes or S9 fractions with NADPH-regenerating systems. Test concentrations spanning 0.1–100 µM, including vehicle controls.
- In vivo: Employ OECD Guideline 489 (micronucleus assay) with doses calibrated to hepatic exposure levels.
- Data Analysis: Apply the linear no-threshold (LNT) model for low-dose extrapolation, using software like BMDS or PROAST .
Q. What strategies mitigate confounding factors in stereoselective metabolism studies?
- Use enantiomerically pure standards synthesized via chiral chromatography.
- Inhibit competing pathways (e.g., glucuronidation with β-glucuronidase inhibitors).
- Quantify sulfate conjugates via LC-MS/MS with selective ion monitoring (SIM) .
Q. Data Contradiction Analysis
Q. Why do some studies report negligible DNA adduct formation by this compound in human cell lines?
Human hepatocytes express lower SULT1A1/1E1 activity compared to rats, reducing metabolic activation. Additionally, human cells exhibit faster adduct repair via nucleotide excision repair (NER). Co-treatment with sulfation cofactors (e.g., PAPS) or siRNA-mediated SULT knockdown can clarify these interspecies differences .
Propiedades
IUPAC Name |
(E)-4-[4-[2-(methylamino)ethoxy]phenyl]-3,4-diphenylbut-3-en-2-ol | |
---|---|---|
Source | PubChem | |
URL | https://pubchem.ncbi.nlm.nih.gov | |
Description | Data deposited in or computed by PubChem | |
InChI |
InChI=1S/C25H27NO2/c1-19(27)24(20-9-5-3-6-10-20)25(21-11-7-4-8-12-21)22-13-15-23(16-14-22)28-18-17-26-2/h3-16,19,26-27H,17-18H2,1-2H3/b25-24- | |
Source | PubChem | |
URL | https://pubchem.ncbi.nlm.nih.gov | |
Description | Data deposited in or computed by PubChem | |
InChI Key |
GREXPZNIZPCGIV-IZHYLOQSSA-N | |
Source | PubChem | |
URL | https://pubchem.ncbi.nlm.nih.gov | |
Description | Data deposited in or computed by PubChem | |
Canonical SMILES |
CC(C(=C(C1=CC=CC=C1)C2=CC=C(C=C2)OCCNC)C3=CC=CC=C3)O | |
Source | PubChem | |
URL | https://pubchem.ncbi.nlm.nih.gov | |
Description | Data deposited in or computed by PubChem | |
Isomeric SMILES |
CC(/C(=C(\C1=CC=CC=C1)/C2=CC=C(C=C2)OCCNC)/C3=CC=CC=C3)O | |
Source | PubChem | |
URL | https://pubchem.ncbi.nlm.nih.gov | |
Description | Data deposited in or computed by PubChem | |
Molecular Formula |
C25H27NO2 | |
Source | PubChem | |
URL | https://pubchem.ncbi.nlm.nih.gov | |
Description | Data deposited in or computed by PubChem | |
DSSTOX Substance ID |
DTXSID401344152 | |
Record name | (E)-4-[4-[2-(methylamino)ethoxy]phenyl]-3,4-diphenylbut-3-en-2-ol | |
Source | EPA DSSTox | |
URL | https://comptox.epa.gov/dashboard/DTXSID401344152 | |
Description | DSSTox provides a high quality public chemistry resource for supporting improved predictive toxicology. | |
Molecular Weight |
373.5 g/mol | |
Source | PubChem | |
URL | https://pubchem.ncbi.nlm.nih.gov | |
Description | Data deposited in or computed by PubChem | |
CAS No. |
162070-61-3 | |
Record name | alpha-Hydroxytamoxifen | |
Source | ChemIDplus | |
URL | https://pubchem.ncbi.nlm.nih.gov/substance/?source=chemidplus&sourceid=0162070613 | |
Description | ChemIDplus is a free, web search system that provides access to the structure and nomenclature authority files used for the identification of chemical substances cited in National Library of Medicine (NLM) databases, including the TOXNET system. | |
Record name | (E)-4-[4-[2-(methylamino)ethoxy]phenyl]-3,4-diphenylbut-3-en-2-ol | |
Source | EPA DSSTox | |
URL | https://comptox.epa.gov/dashboard/DTXSID401344152 | |
Description | DSSTox provides a high quality public chemistry resource for supporting improved predictive toxicology. | |
Retrosynthesis Analysis
AI-Powered Synthesis Planning: Our tool employs the Template_relevance Pistachio, Template_relevance Bkms_metabolic, Template_relevance Pistachio_ringbreaker, Template_relevance Reaxys, Template_relevance Reaxys_biocatalysis model, leveraging a vast database of chemical reactions to predict feasible synthetic routes.
One-Step Synthesis Focus: Specifically designed for one-step synthesis, it provides concise and direct routes for your target compounds, streamlining the synthesis process.
Accurate Predictions: Utilizing the extensive PISTACHIO, BKMS_METABOLIC, PISTACHIO_RINGBREAKER, REAXYS, REAXYS_BIOCATALYSIS database, our tool offers high-accuracy predictions, reflecting the latest in chemical research and data.
Strategy Settings
Precursor scoring | Relevance Heuristic |
---|---|
Min. plausibility | 0.01 |
Model | Template_relevance |
Template Set | Pistachio/Bkms_metabolic/Pistachio_ringbreaker/Reaxys/Reaxys_biocatalysis |
Top-N result to add to graph | 6 |
Feasible Synthetic Routes
Descargo de responsabilidad e información sobre productos de investigación in vitro
Tenga en cuenta que todos los artículos e información de productos presentados en BenchChem están destinados únicamente con fines informativos. Los productos disponibles para la compra en BenchChem están diseñados específicamente para estudios in vitro, que se realizan fuera de organismos vivos. Los estudios in vitro, derivados del término latino "in vidrio", involucran experimentos realizados en entornos de laboratorio controlados utilizando células o tejidos. Es importante tener en cuenta que estos productos no se clasifican como medicamentos y no han recibido la aprobación de la FDA para la prevención, tratamiento o cura de ninguna condición médica, dolencia o enfermedad. Debemos enfatizar que cualquier forma de introducción corporal de estos productos en humanos o animales está estrictamente prohibida por ley. Es esencial adherirse a estas pautas para garantizar el cumplimiento de los estándares legales y éticos en la investigación y experimentación.