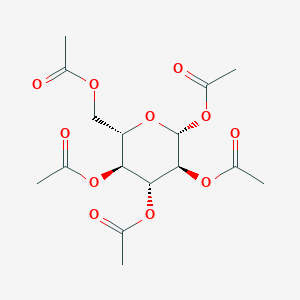
beta-L-glucose pentaacetate
Descripción general
Descripción
Beta-L-glucose pentaacetate: is a derivative of glucose, where all five hydroxyl groups are acetylated. This compound is a sugar derivative with the empirical formula C16H22O11 and a molecular weight of 390.34 g/mol. It is known for its role in stabilizing the amorphous phase of active ingredients in drugs, making it a valuable compound in pharmaceutical sciences .
Métodos De Preparación
Synthetic Routes and Reaction Conditions: Beta-L-glucose pentaacetate can be synthesized through the acetylation of beta-L-glucose. The process typically involves the reaction of beta-L-glucose with acetic anhydride in the presence of a catalyst such as sulfuric acid or pyridine. The reaction is carried out under controlled conditions to ensure complete acetylation of all hydroxyl groups .
Industrial Production Methods: In industrial settings, the preparation of this compound involves similar acetylation reactions but on a larger scale. The use of continuous flow reactors and optimized reaction conditions ensures high yield and purity of the product. The process may also involve purification steps such as recrystallization or chromatography to obtain the desired compound .
Análisis De Reacciones Químicas
Types of Reactions: Beta-L-glucose pentaacetate undergoes various chemical reactions, including:
Hydrolysis: The compound can be hydrolyzed to yield beta-L-glucose and acetic acid.
Glycosylation: this compound can participate in glycosylation reactions to form glycosides.
Oxidation and Reduction: The compound can undergo oxidation to form corresponding carboxylic acids or reduction to form alcohols.
Common Reagents and Conditions:
Hydrolysis: Aqueous acid (e.g., hydrochloric acid) or base (e.g., sodium hydroxide).
Glycosylation: Alcohols and acid catalysts (e.g., sulfuric acid).
Oxidation: Oxidizing agents such as potassium permanganate.
Reduction: Reducing agents such as sodium borohydride
Major Products:
Hydrolysis: Beta-L-glucose and acetic acid.
Glycosylation: Glycosides.
Oxidation: Carboxylic acids.
Reduction: Alcohols
Aplicaciones Científicas De Investigación
Beta-L-glucose pentaacetate has several scientific research applications, including:
Pharmaceutical Sciences: It is used as a stabilizer for the amorphous phase of active pharmaceutical ingredients, enhancing their bioavailability and solubility.
Biochemistry: The compound is used in studies related to insulin release and glucose metabolism.
Organic Synthesis: this compound serves as an intermediate in the synthesis of various glycosides and other sugar derivatives.
Material Science: The compound’s ability to form hydrogen bonds makes it useful in the design of new materials with specific properties.
Mecanismo De Acción
The mechanism of action of beta-L-glucose pentaacetate involves its interaction with specific receptors or enzymes. For instance, its insulinotropic action is attributed to its direct interaction with a receptor that leads to plasma membrane depolarization, induction of electrical activity, and an increase in cytosolic calcium ion concentration. This process is similar to the identification of bitter compounds by taste buds . The compound’s ability to stabilize the amorphous phase of drugs is due to its hydrogen bond acceptor sites, which create an extended hydrogen bond network .
Comparación Con Compuestos Similares
Alpha-D-glucose pentaacetate: Another anomer of glucose pentaacetate with similar properties but different stereochemistry.
Beta-D-glucose pentaacetate: The D-enantiomer of beta-L-glucose pentaacetate, which also has similar applications and properties.
Maltose octaacetate: A disaccharide derivative with multiple acetyl groups, used in similar applications for stabilizing amorphous phases.
Uniqueness: this compound is unique due to its specific stereochemistry, which influences its interaction with biological systems and its ability to stabilize amorphous phases. Its insulinotropic action and role in pharmaceutical sciences make it a valuable compound for research and industrial applications .
Actividad Biológica
Beta-L-glucose pentaacetate is an ester derivative of glucose that has garnered attention for its biological activity, particularly in the context of insulin secretion and metabolic modulation. This article synthesizes findings from diverse sources to provide a comprehensive overview of its biological effects, mechanisms of action, and potential therapeutic applications.
Chemical Structure and Properties
This compound is characterized by the presence of five acetyl groups attached to the glucose molecule. This modification enhances its lipophilicity and may influence its interaction with biological membranes and receptors.
Insulinotropic Action
Mechanism of Action:
Research indicates that this compound stimulates insulin release from pancreatic islets through a mechanism that does not rely on its catabolism. Instead, it appears to interact directly with an unidentified receptor, leading to:
- Plasma membrane depolarization
- Increased intracellular calcium concentration
- Enhanced electrical activity in pancreatic beta cells .
Experimental Evidence:
- A study demonstrated that this compound decreases potassium ion conductance in pancreatic beta cells, which contributes to membrane depolarization and subsequent insulin secretion .
- The compound has been shown to provoke bioelectrical activity in isolated islet cells and induce oscillations in cytosolic calcium levels, further supporting its role as an insulin secretagogue .
Comparative Biological Effects
To understand the relative potency of this compound, it is beneficial to compare it with other glucose derivatives. Below is a summary table highlighting key findings from various studies:
Case Studies
Clinical Implications:
The insulinotropic properties of this compound suggest potential applications in managing conditions like non-insulin-dependent diabetes mellitus (NIDDM). Studies propose that this compound could serve as a novel therapeutic agent due to its unique mechanism of action, which may bypass some limitations associated with traditional glucose-lowering medications .
Research Findings:
- In vitro studies have shown that this compound can enhance insulin release even in the presence of other competing substrates, indicating its potential utility in complex metabolic environments .
- Further investigations into the specific receptor interactions and downstream signaling pathways are needed to elucidate the full therapeutic potential of this compound.
Propiedades
IUPAC Name |
[(2S,3S,4R,5S,6R)-3,4,5,6-tetraacetyloxyoxan-2-yl]methyl acetate | |
---|---|---|
Source | PubChem | |
URL | https://pubchem.ncbi.nlm.nih.gov | |
Description | Data deposited in or computed by PubChem | |
InChI |
InChI=1S/C16H22O11/c1-7(17)22-6-12-13(23-8(2)18)14(24-9(3)19)15(25-10(4)20)16(27-12)26-11(5)21/h12-16H,6H2,1-5H3/t12-,13-,14+,15-,16-/m0/s1 | |
Source | PubChem | |
URL | https://pubchem.ncbi.nlm.nih.gov | |
Description | Data deposited in or computed by PubChem | |
InChI Key |
LPTITAGPBXDDGR-QMHWVQJVSA-N | |
Source | PubChem | |
URL | https://pubchem.ncbi.nlm.nih.gov | |
Description | Data deposited in or computed by PubChem | |
Canonical SMILES |
CC(=O)OCC1C(C(C(C(O1)OC(=O)C)OC(=O)C)OC(=O)C)OC(=O)C | |
Source | PubChem | |
URL | https://pubchem.ncbi.nlm.nih.gov | |
Description | Data deposited in or computed by PubChem | |
Isomeric SMILES |
CC(=O)OC[C@H]1[C@@H]([C@H]([C@@H]([C@H](O1)OC(=O)C)OC(=O)C)OC(=O)C)OC(=O)C | |
Source | PubChem | |
URL | https://pubchem.ncbi.nlm.nih.gov | |
Description | Data deposited in or computed by PubChem | |
Molecular Formula |
C16H22O11 | |
Source | PubChem | |
URL | https://pubchem.ncbi.nlm.nih.gov | |
Description | Data deposited in or computed by PubChem | |
Molecular Weight |
390.34 g/mol | |
Source | PubChem | |
URL | https://pubchem.ncbi.nlm.nih.gov | |
Description | Data deposited in or computed by PubChem | |
Retrosynthesis Analysis
AI-Powered Synthesis Planning: Our tool employs the Template_relevance Pistachio, Template_relevance Bkms_metabolic, Template_relevance Pistachio_ringbreaker, Template_relevance Reaxys, Template_relevance Reaxys_biocatalysis model, leveraging a vast database of chemical reactions to predict feasible synthetic routes.
One-Step Synthesis Focus: Specifically designed for one-step synthesis, it provides concise and direct routes for your target compounds, streamlining the synthesis process.
Accurate Predictions: Utilizing the extensive PISTACHIO, BKMS_METABOLIC, PISTACHIO_RINGBREAKER, REAXYS, REAXYS_BIOCATALYSIS database, our tool offers high-accuracy predictions, reflecting the latest in chemical research and data.
Strategy Settings
Precursor scoring | Relevance Heuristic |
---|---|
Min. plausibility | 0.01 |
Model | Template_relevance |
Template Set | Pistachio/Bkms_metabolic/Pistachio_ringbreaker/Reaxys/Reaxys_biocatalysis |
Top-N result to add to graph | 6 |
Feasible Synthetic Routes
Descargo de responsabilidad e información sobre productos de investigación in vitro
Tenga en cuenta que todos los artículos e información de productos presentados en BenchChem están destinados únicamente con fines informativos. Los productos disponibles para la compra en BenchChem están diseñados específicamente para estudios in vitro, que se realizan fuera de organismos vivos. Los estudios in vitro, derivados del término latino "in vidrio", involucran experimentos realizados en entornos de laboratorio controlados utilizando células o tejidos. Es importante tener en cuenta que estos productos no se clasifican como medicamentos y no han recibido la aprobación de la FDA para la prevención, tratamiento o cura de ninguna condición médica, dolencia o enfermedad. Debemos enfatizar que cualquier forma de introducción corporal de estos productos en humanos o animales está estrictamente prohibida por ley. Es esencial adherirse a estas pautas para garantizar el cumplimiento de los estándares legales y éticos en la investigación y experimentación.