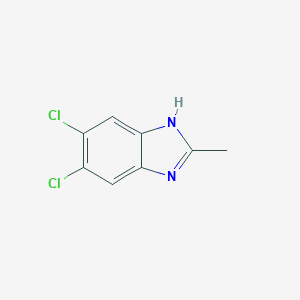
5,6-Dicloro-2-metilbencimidazol
Descripción general
Descripción
5,6-Dichloro-2-methylbenzimidazole: is a chemical compound with the molecular formula C8H6Cl2N2 . It is a derivative of benzimidazole, characterized by the presence of two chlorine atoms at the 5th and 6th positions and a methyl group at the 2nd position of the benzimidazole ring.
Aplicaciones Científicas De Investigación
Chemistry: 5,6-Dichloro-2-methylbenzimidazole is used as a building block in organic synthesis, enabling the creation of more complex molecules for research and industrial applications .
Biology and Medicine: In biological research, this compound has shown potential as an antiparasitic agent and has been studied for its ability to inhibit certain enzymes, such as aminopyrine demethylase .
Materials Science: The compound exhibits ferroelectric properties, making it of interest in the development of advanced materials for electronic applications .
Mecanismo De Acción
Target of Action
The primary target of 5,6-Dichloro-2-methylbenzimidazole is the hydrogen-bonded molecular chain in its crystal structure . The compound exhibits ferroelectricity, which is thought to arise from the positional ordering of protons along this chain of N-H…N hydrogen bonds .
Mode of Action
5,6-Dichloro-2-methylbenzimidazole interacts with its targets through a process known as proton tautomerization . This process allows the compound to be bistable in electric polarity and electrically switchable, even in the crystalline state . The compound allows ferroelectric switching in two dimensions due to its pseudo-tetragonal crystal symmetry .
Biochemical Pathways
The compound affects the polarization-electric field (P-E) hysteresis . This process involves the spontaneous electric polarization that can be reversibly inverted by reversing the electric field . The compound exhibits a high electric polarization ranging from 5 to 10 mCcm^2 at room temperature .
Result of Action
The compound exhibits ferroelectricity above room temperature . This ferroelectricity is thermally robust up to around 400 K . The compound undergoes a first-order phase transition at around 400 K, as detected by a discontinuity in the temperature dependence of NQR frequencies .
Action Environment
The action of 5,6-Dichloro-2-methylbenzimidazole is influenced by temperature. The compound undergoes an unusual symmetry-lowering transition on warming from orthorhombic Pca21 to monoclinic Pc . The high-temperature phase of the compound remains polar .
Análisis Bioquímico
Biochemical Properties
It is known that the compound is chemically stable and can easily bind molecules into a dipolar chain
Cellular Effects
Given its ability to bind molecules into a dipolar chain, it may influence cell function by impacting cell signaling pathways, gene expression, and cellular metabolism .
Molecular Mechanism
It is thought to exert its effects at the molecular level through proton tautomerization, which could involve binding interactions with biomolecules, enzyme inhibition or activation, and changes in gene expression .
Temporal Effects in Laboratory Settings
It is known that the compound undergoes a symmetry-lowering transition on warming from orthorhombic Pca21 (T ≲ 400 K) to monoclinic Pc . This suggests that the compound’s effects may change over time in response to temperature changes.
Métodos De Preparación
Synthetic Routes and Reaction Conditions: The synthesis of 5,6-Dichloro-2-methylbenzimidazole typically involves the reaction of o-phenylenediamine with 5,6-dichloro-2-methylbenzaldehyde under acidic conditions. The reaction proceeds through a cyclization process, forming the benzimidazole ring .
Industrial Production Methods: Industrial production methods for 5,6-Dichloro-2-methylbenzimidazole may involve similar synthetic routes but are optimized for large-scale production. This includes the use of continuous flow reactors and efficient purification techniques to ensure high yield and purity of the final product .
Análisis De Reacciones Químicas
Types of Reactions: 5,6-Dichloro-2-methylbenzimidazole undergoes various chemical reactions, including:
Substitution Reactions: The chlorine atoms at the 5th and 6th positions can be substituted with other functional groups through nucleophilic substitution reactions.
Oxidation and Reduction Reactions: The compound can undergo oxidation and reduction reactions, altering its chemical structure and properties.
Common Reagents and Conditions:
Nucleophilic Substitution: Common reagents include sodium hydroxide (NaOH) and potassium carbonate (K2CO3) under reflux conditions.
Oxidation: Reagents such as potassium permanganate (KMnO4) or hydrogen peroxide (H2O2) can be used.
Reduction: Reducing agents like sodium borohydride (NaBH4) or lithium aluminum hydride (LiAlH4) are commonly employed.
Major Products Formed: The major products formed from these reactions depend on the specific reagents and conditions used. For example, nucleophilic substitution can yield various substituted benzimidazoles, while oxidation and reduction reactions can lead to different oxidation states of the compound .
Comparación Con Compuestos Similares
5,6-Dimethylbenzimidazole: A derivative with two methyl groups instead of chlorine atoms, known for its role in the biosynthesis of vitamin B12.
2-Methylbenzimidazole: Lacks the chlorine atoms, making it less reactive in certain chemical reactions.
Uniqueness: 5,6-Dichloro-2-methylbenzimidazole is unique due to the presence of chlorine atoms, which enhance its reactivity and enable a wider range of chemical modifications. Its ferroelectric properties also distinguish it from other benzimidazole derivatives .
Actividad Biológica
5,6-Dichloro-2-methylbenzimidazole (DC-MBI) is a compound that has garnered attention due to its diverse biological activities, particularly in the fields of medicinal chemistry and pharmacology. This article reviews the synthesis, biological effects, and mechanisms of action associated with DC-MBI, supported by relevant case studies and research findings.
- Molecular Formula : CHClN
- Molecular Weight : 201.05 g/mol
- CAS Number : 6478-79-1
- Physical State : Solid at room temperature
- Purity : >98.0% (GC)
DC-MBI is characterized by its two chlorine substituents and a methyl group on the benzimidazole ring, which contribute to its unique chemical properties and biological activities.
Synthesis
DC-MBI can be synthesized through various methods involving chlorination and methylation of benzimidazole derivatives. A common synthetic route involves the use of chlorinating agents followed by methylating agents under controlled conditions to yield high yields of the desired compound .
1. Urease Inhibition
A significant area of research has focused on the urease inhibitory activity of DC-MBI and its derivatives. In a study, a series of 5,6-dichloro-2-methylbenzimidazole derivatives were synthesized and evaluated for their urease inhibition potential. The most potent compound exhibited an IC value of 0.0294 µM, significantly lower than that of thiourea (IC = 0.5117 µM), indicating a strong inhibitory effect on urease activity .
2. Antinociceptive Effects
Research has demonstrated that DC-MBI acts as an antagonist at the P2X3 receptor, which is implicated in pain pathways. In neuropathic pain models involving nerve ligation or chemotherapy-induced pain in rats, DC-MBI showed significant anti-nociceptive effects. It increased the mechanical withdrawal threshold, suggesting its potential as a therapeutic agent for managing neuropathic pain .
3. Ferroelectric Properties
Beyond its pharmacological applications, DC-MBI has been studied for its ferroelectric properties. It undergoes a phase transition from orthorhombic to monoclinic structure at elevated temperatures while retaining polar characteristics, making it a candidate for applications in electronic devices .
The biological activities of DC-MBI are largely attributed to its interaction with specific receptors and enzymes:
- P2X3 Receptor Antagonism : By inhibiting P2X3 receptors, DC-MBI reduces nociceptive signaling pathways, thus alleviating pain.
- Urease Binding : Molecular docking studies have elucidated how DC-MBI binds to urease active sites, disrupting its enzymatic function.
Case Studies
- Study on Urease Inhibition :
- Antinociceptive Activity in Animal Models :
Propiedades
IUPAC Name |
5,6-dichloro-2-methyl-1H-benzimidazole | |
---|---|---|
Source | PubChem | |
URL | https://pubchem.ncbi.nlm.nih.gov | |
Description | Data deposited in or computed by PubChem | |
InChI |
InChI=1S/C8H6Cl2N2/c1-4-11-7-2-5(9)6(10)3-8(7)12-4/h2-3H,1H3,(H,11,12) | |
Source | PubChem | |
URL | https://pubchem.ncbi.nlm.nih.gov | |
Description | Data deposited in or computed by PubChem | |
InChI Key |
WMOBNOCVMZFPEN-UHFFFAOYSA-N | |
Source | PubChem | |
URL | https://pubchem.ncbi.nlm.nih.gov | |
Description | Data deposited in or computed by PubChem | |
Canonical SMILES |
CC1=NC2=CC(=C(C=C2N1)Cl)Cl | |
Source | PubChem | |
URL | https://pubchem.ncbi.nlm.nih.gov | |
Description | Data deposited in or computed by PubChem | |
Molecular Formula |
C8H6Cl2N2 | |
Source | PubChem | |
URL | https://pubchem.ncbi.nlm.nih.gov | |
Description | Data deposited in or computed by PubChem | |
DSSTOX Substance ID |
DTXSID10345959 | |
Record name | 5,6-Dichloro-2-methylbenzimidazole | |
Source | EPA DSSTox | |
URL | https://comptox.epa.gov/dashboard/DTXSID10345959 | |
Description | DSSTox provides a high quality public chemistry resource for supporting improved predictive toxicology. | |
Molecular Weight |
201.05 g/mol | |
Source | PubChem | |
URL | https://pubchem.ncbi.nlm.nih.gov | |
Description | Data deposited in or computed by PubChem | |
CAS No. |
6478-79-1 | |
Record name | 5,6-Dichloro-2-methylbenzimidazole | |
Source | EPA DSSTox | |
URL | https://comptox.epa.gov/dashboard/DTXSID10345959 | |
Description | DSSTox provides a high quality public chemistry resource for supporting improved predictive toxicology. | |
Record name | 5,6-Dichloro-2-methylbenzimidazole | |
Source | European Chemicals Agency (ECHA) | |
URL | https://echa.europa.eu/information-on-chemicals | |
Description | The European Chemicals Agency (ECHA) is an agency of the European Union which is the driving force among regulatory authorities in implementing the EU's groundbreaking chemicals legislation for the benefit of human health and the environment as well as for innovation and competitiveness. | |
Explanation | Use of the information, documents and data from the ECHA website is subject to the terms and conditions of this Legal Notice, and subject to other binding limitations provided for under applicable law, the information, documents and data made available on the ECHA website may be reproduced, distributed and/or used, totally or in part, for non-commercial purposes provided that ECHA is acknowledged as the source: "Source: European Chemicals Agency, http://echa.europa.eu/". Such acknowledgement must be included in each copy of the material. ECHA permits and encourages organisations and individuals to create links to the ECHA website under the following cumulative conditions: Links can only be made to webpages that provide a link to the Legal Notice page. | |
Synthesis routes and methods
Procedure details
Retrosynthesis Analysis
AI-Powered Synthesis Planning: Our tool employs the Template_relevance Pistachio, Template_relevance Bkms_metabolic, Template_relevance Pistachio_ringbreaker, Template_relevance Reaxys, Template_relevance Reaxys_biocatalysis model, leveraging a vast database of chemical reactions to predict feasible synthetic routes.
One-Step Synthesis Focus: Specifically designed for one-step synthesis, it provides concise and direct routes for your target compounds, streamlining the synthesis process.
Accurate Predictions: Utilizing the extensive PISTACHIO, BKMS_METABOLIC, PISTACHIO_RINGBREAKER, REAXYS, REAXYS_BIOCATALYSIS database, our tool offers high-accuracy predictions, reflecting the latest in chemical research and data.
Strategy Settings
Precursor scoring | Relevance Heuristic |
---|---|
Min. plausibility | 0.01 |
Model | Template_relevance |
Template Set | Pistachio/Bkms_metabolic/Pistachio_ringbreaker/Reaxys/Reaxys_biocatalysis |
Top-N result to add to graph | 6 |
Feasible Synthetic Routes
Q1: What makes 5,6-Dichloro-2-methylbenzimidazole special in the realm of ferroelectric materials?
A1: Unlike traditional inorganic ferroelectrics, 5,6-Dichloro-2-methylbenzimidazole belongs to a class of materials known as molecular ferroelectrics. These materials are organic and derive their ferroelectric properties from the ordering of protons within hydrogen bonds. [] This offers advantages like low processing temperatures, mechanical flexibility, and environmental friendliness compared to their inorganic counterparts. []
Q2: How does the crystal structure of 5,6-Dichloro-2-methylbenzimidazole change with temperature, and how does this relate to its ferroelectric behavior?
A2: 5,6-Dichloro-2-methylbenzimidazole exhibits an intriguing characteristic: it undergoes a symmetry-lowering transition upon heating. At lower temperatures (below ~400K), it exists in an orthorhombic Pca21 phase. As the temperature rises, it transitions to a monoclinic Pc phase. Interestingly, both phases remain polar, contributing to its above-room-temperature ferroelectricity. [] This unusual transition was further confirmed by observing a significant difference in 35Cl Nuclear Quadruple Resonance (NQR) frequencies between the two phases. []
Q3: Can we predict the ferroelectric behavior of 5,6-Dichloro-2-methylbenzimidazole computationally?
A3: Yes, van der Waals density-functional theory (vdW-DFT) calculations accurately predict the crystal structure parameters and spontaneous polarization values of 5,6-Dichloro-2-methylbenzimidazole, showing good agreement with experimental findings. [] This computational approach allows researchers to further investigate electromechanical responses like piezoelectricity under various conditions.
Q4: How does the microstructure of thin films impact the macroscopic ferroelectric properties of 5,6-Dichloro-2-methylbenzimidazole?
A4: Research shows that the macroscopic ferroelectric behavior of 5,6-Dichloro-2-methylbenzimidazole thin films is highly dependent on their microstructure. Dense spherulite formations within the film promote macroscopic polarization switching, while porous microstructures with randomly oriented nanograins hinder this behavior. [] This highlights the importance of controlled film fabrication methods for achieving desired ferroelectric properties.
Q5: Can 5,6-Dichloro-2-methylbenzimidazole be utilized for terahertz wave generation?
A5: Indeed, research has demonstrated the generation of terahertz radiation from 5,6-Dichloro-2-methylbenzimidazole upon excitation with femtosecond laser pulses. [, ] This terahertz emission is attributed to polarization modulations caused by Raman- and infrared-active phonon modes within the material. This finding opens up exciting possibilities for its application in terahertz technologies. [, ]
Q6: What are the future directions of research on 5,6-Dichloro-2-methylbenzimidazole?
A6: Further research on 5,6-Dichloro-2-methylbenzimidazole is crucial for exploring its full potential. This includes investigating:
- New synthesis methods: Optimizing current synthesis routes [] and exploring new approaches for large-scale production are essential.
- Structure-property relationships: Studying the impact of chemical modifications on its ferroelectric and other properties [] will guide the development of tailored materials.
- Device fabrication and integration: Investigating thin film deposition techniques and device architectures [] will pave the way for real-world applications in sensors, actuators, and other electronic devices.
- Understanding the mechanism of THz generation: Further exploring the interplay between phonon modes and terahertz radiation [, ] can unlock novel functionalities and applications in terahertz technology.
Descargo de responsabilidad e información sobre productos de investigación in vitro
Tenga en cuenta que todos los artículos e información de productos presentados en BenchChem están destinados únicamente con fines informativos. Los productos disponibles para la compra en BenchChem están diseñados específicamente para estudios in vitro, que se realizan fuera de organismos vivos. Los estudios in vitro, derivados del término latino "in vidrio", involucran experimentos realizados en entornos de laboratorio controlados utilizando células o tejidos. Es importante tener en cuenta que estos productos no se clasifican como medicamentos y no han recibido la aprobación de la FDA para la prevención, tratamiento o cura de ninguna condición médica, dolencia o enfermedad. Debemos enfatizar que cualquier forma de introducción corporal de estos productos en humanos o animales está estrictamente prohibida por ley. Es esencial adherirse a estas pautas para garantizar el cumplimiento de los estándares legales y éticos en la investigación y experimentación.