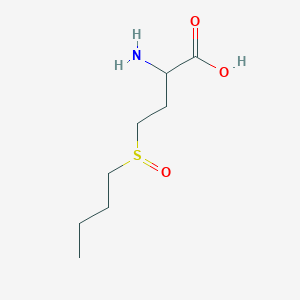
D,L-Butionina Sulfóxido
Descripción general
Descripción
It is a potent inhibitor of glutamine synthetase, an enzyme that catalyzes the conversion of glutamate and ammonia to glutamine. This compound has been studied for its potential therapeutic and toxic effects.
Aplicaciones Científicas De Investigación
D,L-Buthionine Sulfoxide has several scientific research applications:
Chemistry: Used as a reagent in organic synthesis and as a building block for more complex molecules.
Biology: Studied for its role as an inhibitor of glutamine synthetase, which is important in nitrogen metabolism.
Medicine: Investigated for its potential therapeutic effects, including its role in modulating glutamine levels in the body.
Industry: Used in the production of various chemical intermediates and as a research tool in biochemical studies.
Mecanismo De Acción
Target of Action
The primary target of D,L-Buthionine Sulfoxide is γ-glutamylcysteine synthetase , an essential enzyme for the synthesis of glutathione (GSH) . This enzyme plays a crucial role in maintaining the balance of reactive oxygen species (ROS) in cells .
Mode of Action
D,L-Buthionine Sulfoxide acts as an irreversible inhibitor of γ-glutamylcysteine synthetase . By inhibiting this enzyme, it disrupts the synthesis of GSH, leading to a decrease in cellular GSH levels . This results in an increase in the level of ROS within the cell .
Biochemical Pathways
The inhibition of γ-glutamylcysteine synthetase by D,L-Buthionine Sulfoxide affects the glutathione synthesis pathway . This leads to a decrease in the levels of GSH, a critical antioxidant in cells. The reduction in GSH levels increases the level of ROS, leading to oxidative stress .
Pharmacokinetics
It has been shown to have biexponential elimination and differential clearance between l,r and l,s stereoisomers .
Result of Action
The increase in ROS levels due to the action of D,L-Buthionine Sulfoximine can lead to DNA damage and other oxidative damages . This can result in the arrest of the cell cycle, leading to cell death . In some cases, this can enhance the sensitivity of cells to other treatments, such as chemotherapy .
Action Environment
The action of D,L-Buthionine Sulfoximine can be influenced by various environmental factors. For example, the presence of other antioxidants in the cell can counteract the pro-oxidant conditions induced by D,L-Buthionine Sulfoximine . Additionally, the compound’s action can be influenced by the metabolic state of the cell and the presence of other compounds .
Análisis Bioquímico
Biochemical Properties
D,L-Buthionine Sulfoxide plays a significant role in biochemical reactions by inhibiting the enzyme gamma-glutamylcysteine synthetase . This enzyme is crucial for the synthesis of glutathione, a powerful antioxidant in cells . By inhibiting this enzyme, D,L-Buthionine Sulfoxide effectively reduces the levels of glutathione in cells .
Cellular Effects
The reduction of glutathione levels by D,L-Buthionine Sulfoxide has profound effects on various types of cells and cellular processes. Glutathione is involved in several cellular functions, including cell signaling pathways, gene expression, and cellular metabolism . Therefore, the reduction of glutathione levels by D,L-Buthionine Sulfoxide can influence these cellular functions.
Molecular Mechanism
D,L-Buthionine Sulfoxide exerts its effects at the molecular level primarily through its inhibition of gamma-glutamylcysteine synthetase . This inhibition leads to a decrease in the synthesis of glutathione, thereby affecting various cellular and molecular processes that depend on glutathione .
Temporal Effects in Laboratory Settings
In laboratory settings, the effects of D,L-Buthionine Sulfoxide can change over time. For instance, it has been observed that D,L-Buthionine Sulfoxide can lead to a significant reduction in glutathione levels in cells within a certain period .
Dosage Effects in Animal Models
The effects of D,L-Buthionine Sulfoxide can vary with different dosages in animal models. For instance, it has been observed that D,L-Buthionine Sulfoxide can lead to a significant reduction in glutathione levels in mice when administered at certain dosages .
Metabolic Pathways
D,L-Buthionine Sulfoxide is involved in the metabolic pathway of glutathione synthesis. It interacts with the enzyme gamma-glutamylcysteine synthetase, which is crucial for the synthesis of glutathione .
Métodos De Preparación
Synthetic Routes and Reaction Conditions
The synthesis of D,L-Buthionine Sulfoxide can be achieved through various synthetic routes. One common method involves the oxidation of L-Buthionine to form L-Buthionine Sulfoxide. This reaction typically uses oxidizing agents such as hydrogen peroxide or m-chloroperbenzoic acid under controlled conditions .
Industrial Production Methods
Industrial production of D,L-Buthionine Sulfoxide may involve large-scale oxidation processes. The choice of oxidizing agent and reaction conditions can be optimized to ensure high yield and purity of the final product .
Análisis De Reacciones Químicas
Types of Reactions
D,L-Buthionine Sulfoxide undergoes various chemical reactions, including:
Oxidation: The sulfoxide group can be further oxidized to sulfone using strong oxidizing agents.
Reduction: The sulfoxide group can be reduced back to the sulfide using reducing agents such as lithium aluminum hydride.
Substitution: The amino group can participate in substitution reactions with electrophiles.
Common Reagents and Conditions
Oxidation: Hydrogen peroxide, m-chloroperbenzoic acid.
Reduction: Lithium aluminum hydride.
Substitution: Electrophiles such as alkyl halides.
Major Products Formed
Oxidation: Formation of sulfone derivatives.
Reduction: Formation of sulfide derivatives.
Substitution: Formation of substituted amino acid derivatives.
Comparación Con Compuestos Similares
Similar Compounds
L-Buthionine Sulfoximine: Another inhibitor of glutamine synthetase, but with a different chemical structure.
2-Amino-4-(hydroxymethyl-phosphinyl)butanoic acid: A compound with similar inhibitory effects on glutamine synthetase.
Uniqueness
D,L-Buthionine Sulfoxide is unique due to its specific sulfoxide group, which imparts distinct chemical and biological properties. Its ability to inhibit glutamine synthetase makes it a valuable tool in biochemical research and potential therapeutic applications .
Actividad Biológica
D,L-Buthionine sulfoxide (BSO) is a synthetic compound primarily recognized for its role as an inhibitor of glutathione biosynthesis. This article explores the biological activity of BSO, focusing on its mechanisms, effects on various cell types, and potential therapeutic applications, supported by data tables and case studies.
BSO functions by inhibiting gamma-glutamylcysteine synthetase (γ-GCS), the enzyme responsible for the first step in glutathione synthesis. This inhibition leads to a significant reduction in intracellular glutathione levels, which affects numerous metabolic pathways and cellular functions. Notably, BSO acts as a competitive inhibitor, binding to the active site of γ-GCS and preventing substrate access. Studies indicate that concentrations as low as 10 μM can induce complete inhibition of this enzyme.
Biological Effects
The depletion of glutathione due to BSO treatment has been linked to various biological effects:
- Cell Cycle Arrest : BSO causes arrest in the G2/M phase of the cell cycle, leading to increased apoptosis in cancer cells.
- Increased Oxidative Stress : By reducing antioxidant defenses, BSO enhances oxidative stress, making it a valuable tool in studying oxidative damage in various diseases, including cancer and neurodegenerative disorders .
- Potentiation of Chemotherapy : BSO has been shown to sensitize tumor cells to chemotherapeutic agents by depleting glutathione, thereby enhancing drug efficacy .
1. Cancer Treatment Efficacy
A study evaluated the combined effects of BSO and azathioprine on HepG2 cells (human liver cancer cells). The results indicated that pretreatment with BSO significantly enhanced the cytotoxicity of azathioprine, with a dose modification factor (DMF) of 3.4 for melphalan and 3.3 for nitrogen mustard. The increased cytotoxicity correlated with enhanced DNA cross-linking, indicating that BSO effectively potentiates the effects of DNA-damaging agents .
2. Hypertension Induction
Research involving male Wistar albino rats demonstrated that administration of BSO led to significant increases in blood pressure when coadministered with N-nitro-L-arginine (L-NNA). This study highlighted the role of oxidative stress induced by BSO in altering renal function and blood pressure regulation .
3. Antiparasitic Activity
In vivo studies have shown that BSO has antiparasitic effects against Trypanosoma brucei by depleting intratrypanosomal glutathione, leading to oxidative stress and elimination of the parasite from infected mice at doses of 4 mmol/kg .
Comparative Analysis with Similar Compounds
Compound Name | Mechanism | Unique Features |
---|---|---|
L-Buthionine Sulfoximine | Inhibits γ-GCS | More potent than D,L-BSO |
N-acetylcysteine | Precursor for glutathione synthesis | Acts as a reducing agent and antioxidant |
Glutathione | Natural antioxidant | Plays a critical role in detoxification |
Cysteamine | Precursor for cysteine | Involved in various metabolic pathways |
D,L-Buthionine sulfoxide is distinct due to its specific role as a competitive inhibitor of γ-GCS, leading to targeted depletion of glutathione without directly providing antioxidant properties like N-acetylcysteine or glutathione itself.
Propiedades
IUPAC Name |
2-amino-4-butylsulfinylbutanoic acid | |
---|---|---|
Source | PubChem | |
URL | https://pubchem.ncbi.nlm.nih.gov | |
Description | Data deposited in or computed by PubChem | |
InChI |
InChI=1S/C8H17NO3S/c1-2-3-5-13(12)6-4-7(9)8(10)11/h7H,2-6,9H2,1H3,(H,10,11) | |
Source | PubChem | |
URL | https://pubchem.ncbi.nlm.nih.gov | |
Description | Data deposited in or computed by PubChem | |
InChI Key |
KOGSTWSVCBVYHO-UHFFFAOYSA-N | |
Source | PubChem | |
URL | https://pubchem.ncbi.nlm.nih.gov | |
Description | Data deposited in or computed by PubChem | |
Canonical SMILES |
CCCCS(=O)CCC(C(=O)O)N | |
Source | PubChem | |
URL | https://pubchem.ncbi.nlm.nih.gov | |
Description | Data deposited in or computed by PubChem | |
Molecular Formula |
C8H17NO3S | |
Source | PubChem | |
URL | https://pubchem.ncbi.nlm.nih.gov | |
Description | Data deposited in or computed by PubChem | |
DSSTOX Substance ID |
DTXSID80326553 | |
Record name | NSC601361 | |
Source | EPA DSSTox | |
URL | https://comptox.epa.gov/dashboard/DTXSID80326553 | |
Description | DSSTox provides a high quality public chemistry resource for supporting improved predictive toxicology. | |
Molecular Weight |
207.29 g/mol | |
Source | PubChem | |
URL | https://pubchem.ncbi.nlm.nih.gov | |
Description | Data deposited in or computed by PubChem | |
CAS No. |
98487-33-3 | |
Record name | NSC601361 | |
Source | EPA DSSTox | |
URL | https://comptox.epa.gov/dashboard/DTXSID80326553 | |
Description | DSSTox provides a high quality public chemistry resource for supporting improved predictive toxicology. | |
Descargo de responsabilidad e información sobre productos de investigación in vitro
Tenga en cuenta que todos los artículos e información de productos presentados en BenchChem están destinados únicamente con fines informativos. Los productos disponibles para la compra en BenchChem están diseñados específicamente para estudios in vitro, que se realizan fuera de organismos vivos. Los estudios in vitro, derivados del término latino "in vidrio", involucran experimentos realizados en entornos de laboratorio controlados utilizando células o tejidos. Es importante tener en cuenta que estos productos no se clasifican como medicamentos y no han recibido la aprobación de la FDA para la prevención, tratamiento o cura de ninguna condición médica, dolencia o enfermedad. Debemos enfatizar que cualquier forma de introducción corporal de estos productos en humanos o animales está estrictamente prohibida por ley. Es esencial adherirse a estas pautas para garantizar el cumplimiento de los estándares legales y éticos en la investigación y experimentación.