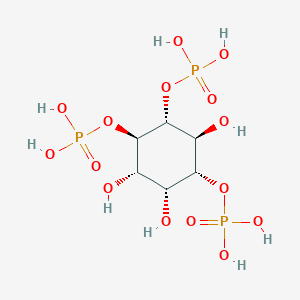
d-Mio-inositol-1,4,5-trifosfato
Descripción general
Descripción
Inositol 1, 4, 5-trisphosphate, also known as 1, 4, 5-insp3 or IP3, belongs to the class of organic compounds known as inositol phosphates. Inositol phosphates are compounds containing a phosphate group attached to an inositol (or cyclohexanehexol) moiety. Inositol 1, 4, 5-trisphosphate is soluble (in water) and an extremely strong acidic compound (based on its pKa). Inositol 1, 4, 5-trisphosphate has been found in human epidermis, pancreas and spleen tissues. Within the cell, inositol 1, 4, 5-trisphosphate is primarily located in the cytoplasm and nucleus. Inositol 1, 4, 5-trisphosphate exists in all eukaryotes, ranging from yeast to humans. Inositol 1, 4, 5-trisphosphate and DG(14:0/14:1(9Z)/0:0) can be biosynthesized from pip2(16:0/20:3(8Z, 11Z, 14Z)); which is catalyzed by the enzyme 1-phosphatidylinositol 4, 5-bisphosphate phosphodiesterase beta-1. In humans, inositol 1, 4, 5-trisphosphate is involved in the labetalol action pathway, the bevantolol action pathway, the tripelennamine H1-antihistamine action pathway, and the penbutolol action pathway. Inositol 1, 4, 5-trisphosphate is also involved in several metabolic disorders, some of which include thenyldiamine H1-antihistamine action pathway, the phenytoin (antiarrhythmic) action pathway, the lidocaine (antiarrhythmic) action pathway, and the thiazinamium H1-antihistamine action pathway.
1D-myo-inositol 1,4,5-trisphosphate is a myo-inositol trisphosphate. It has a role as a mouse metabolite. It is a conjugate acid of a 1D-myo-inositol 1,4,5-trisphosphate(6-).
Intracellular messenger formed by the action of phospholipase C on phosphatidylinositol 4,5-bisphosphate, which is one of the phospholipids that make up the cell membrane. Inositol 1,4,5-trisphosphate is released into the cytoplasm where it releases calcium ions from internal stores within the cell's endoplasmic reticulum. These calcium ions stimulate the activity of B kinase or calmodulin.
Aplicaciones Científicas De Investigación
- d-InsP₃ actúa como un segundo mensajero, movilizando iones de calcio (Ca²⁺) de las reservas intracelulares. Se forma a través de la hidrólisis enzimática del fosfatidilinositol-4,5-bisfosfato .
- Investigar el impacto de d-InsP₃ en las vías de señalización de la insulina puede proporcionar información sobre los trastornos metabólicos .
- Investigar su impacto en la proliferación celular, la apoptosis y la angiogénesis proporciona posibles objetivos terapéuticos .
Señalización de calcio y liberación intracelular
Trastornos metabólicos y sensibilidad a la insulina
Cáncer y proliferación celular
Fertilidad y salud reproductiva
Estas aplicaciones destacan el papel multifacético del d-Mio-inositol-1,4,5-trifosfato en la salud y la enfermedad. La investigación adicional puede descubrir estrategias terapéuticas e intervenciones clínicas adicionales . Si desea más detalles sobre cualquier área específica, ¡no dude en preguntar!
Mecanismo De Acción
Target of Action
D-Myo-Inositol-1,4,5-triphosphate (IP3) is a second messenger that plays a crucial role in cellular signaling . Its primary targets are the IP3 receptors located on the membrane of the endoplasmic reticulum . These receptors are calcium channels, and their primary role is to regulate the release of calcium ions (Ca2+) from the endoplasmic reticulum .
Mode of Action
IP3 is produced in cells by the action of phospholipase C on phosphatidylinositol 4,5-bisphosphate . Once formed, IP3 binds to its receptors (IP3R) on the endoplasmic reticulum . This binding triggers the opening of the calcium channels and the release of Ca2+ into the cytoplasm .
Biochemical Pathways
The release of Ca2+ into the cytoplasm triggers various downstream effects, influencing numerous biochemical pathways. For instance, the increase in cytoplasmic Ca2+ can activate calmodulin and protein kinase C, which are integral to processes such as cell growth, survival, and metabolism .
Result of Action
The primary result of IP3 action is the mobilization of intracellular Ca2+ stores . This increase in cytoplasmic Ca2+ concentration can lead to various cellular responses, including muscle contraction, secretion of hormones, and activation of enzymes .
Análisis Bioquímico
Biochemical Properties
d-Myo-inositol-1,4,5-triphosphate is involved in several biochemical reactions, primarily as a second messenger that stimulates the release of calcium from the endoplasmic reticulum. It interacts with the inositol 1,4,5-triphosphate receptor, a calcium channel located on the membrane of the endoplasmic reticulum. Upon binding to this receptor, d-Myo-inositol-1,4,5-triphosphate induces the opening of the calcium channel, leading to an increase in cytoplasmic calcium levels . This interaction is crucial for the regulation of various cellular functions, including muscle contraction, secretion, metabolism, and cell growth.
Cellular Effects
d-Myo-inositol-1,4,5-triphosphate has profound effects on various cell types and cellular processes. It plays a pivotal role in cell signaling pathways by modulating intracellular calcium levels. The increase in cytoplasmic calcium triggered by d-Myo-inositol-1,4,5-triphosphate influences numerous cellular functions, such as gene expression, enzyme activity, and cellular metabolism . For instance, in neurons, it is involved in neurotransmitter release, while in muscle cells, it regulates contraction. Additionally, d-Myo-inositol-1,4,5-triphosphate is implicated in processes like cell proliferation and apoptosis.
Molecular Mechanism
The molecular mechanism of d-Myo-inositol-1,4,5-triphosphate involves its binding to the inositol 1,4,5-triphosphate receptor on the endoplasmic reticulum membrane. This binding induces a conformational change in the receptor, leading to the opening of the calcium channel and the subsequent release of calcium ions into the cytoplasm . The elevated calcium levels activate various calcium-dependent proteins and enzymes, such as calmodulin and protein kinase C, which further propagate the signal and elicit specific cellular responses. Additionally, d-Myo-inositol-1,4,5-triphosphate can influence gene expression by activating transcription factors that are sensitive to changes in intracellular calcium levels.
Temporal Effects in Laboratory Settings
In laboratory settings, the effects of d-Myo-inositol-1,4,5-triphosphate can vary over time. The compound is relatively stable under physiological conditions but can degrade over extended periods or under specific experimental conditions . Studies have shown that the initial release of calcium triggered by d-Myo-inositol-1,4,5-triphosphate is rapid, occurring within seconds to minutes. Prolonged exposure to d-Myo-inositol-1,4,5-triphosphate can lead to desensitization of the inositol 1,4,5-triphosphate receptor, reducing its responsiveness to subsequent stimuli. Long-term effects of d-Myo-inositol-1,4,5-triphosphate on cellular function have been observed in both in vitro and in vivo studies, including alterations in cell proliferation and apoptosis.
Dosage Effects in Animal Models
The effects of d-Myo-inositol-1,4,5-triphosphate in animal models are dose-dependent. At low doses, d-Myo-inositol-1,4,5-triphosphate effectively stimulates calcium release and modulates cellular functions without causing significant adverse effects . At higher doses, the compound can induce excessive calcium release, leading to cytotoxicity and cell death. Threshold effects have been observed, where a minimal concentration of d-Myo-inositol-1,4,5-triphosphate is required to elicit a measurable response. Toxic effects at high doses include disruption of cellular homeostasis, oxidative stress, and activation of apoptotic pathways.
Metabolic Pathways
d-Myo-inositol-1,4,5-triphosphate is involved in several metabolic pathways, primarily related to its role as a second messenger in calcium signaling . It is produced by the enzymatic hydrolysis of phosphatidylinositol-4,5-bisphosphate by phospholipase C. Once formed, d-Myo-inositol-1,4,5-triphosphate can be further phosphorylated to produce other inositol phosphates, such as inositol 1,3,4,5-tetrakisphosphate, which also participate in cellular signaling. The metabolism of d-Myo-inositol-1,4,5-triphosphate is tightly regulated by various enzymes, including inositol polyphosphate 5-phosphatases and inositol trisphosphate 3-kinases, which modulate its levels and activity within the cell.
Transport and Distribution
d-Myo-inositol-1,4,5-triphosphate is transported and distributed within cells and tissues through specific transporters and binding proteins . It is primarily localized in the cytoplasm, where it interacts with its receptor on the endoplasmic reticulum. The distribution of d-Myo-inositol-1,4,5-triphosphate within the cell is influenced by its production and degradation rates, as well as its binding affinity to various intracellular proteins. Additionally, the compound can diffuse through the cytoplasm to reach its target sites, ensuring a rapid and efficient response to extracellular signals.
Subcellular Localization
The subcellular localization of d-Myo-inositol-1,4,5-triphosphate is predominantly in the cytoplasm, where it exerts its effects on calcium signaling . It is produced at the plasma membrane and diffuses through the cytoplasm to bind to its receptor on the endoplasmic reticulum. The activity and function of d-Myo-inositol-1,4,5-triphosphate are influenced by its localization, as it needs to be in proximity to its receptor to effectively stimulate calcium release. Post-translational modifications and targeting signals may also play a role in directing d-Myo-inositol-1,4,5-triphosphate to specific cellular compartments or organelles.
Actividad Biológica
d-Myo-inositol-1,4,5-triphosphate (Ins(1,4,5)P3) is a crucial second messenger involved in various cellular processes, particularly in the mobilization of calcium ions (Ca²⁺) from intracellular stores. This compound plays a significant role in signal transduction pathways linked to growth factors, hormones, and neurotransmitters. Understanding its biological activity is essential for elucidating its functions in physiological and pathological contexts.
Structure and Synthesis
Ins(1,4,5)P3 is synthesized from phosphatidylinositol-4,5-bisphosphate (PIP2) through the action of phospholipase C (PLC). The enzymatic hydrolysis of PIP2 generates Ins(1,4,5)P3 and diacylglycerol (DAG), both of which serve as important signaling molecules. The structure of Ins(1,4,5)P3 features a myo-inositol ring with three phosphate groups attached at the 1, 4, and 5 positions.
Ins(1,4,5)P3 primarily exerts its effects by binding to inositol trisphosphate receptors (IP3Rs) located on the endoplasmic reticulum (ER). This interaction triggers the release of Ca²⁺ into the cytosol, leading to various downstream effects including:
- Muscle Contraction : Ca²⁺ release stimulates muscle contraction through activation of contractile proteins.
- Neurotransmitter Release : In neurons, Ins(1,4,5)P3-mediated Ca²⁺ release facilitates neurotransmitter release at synapses.
- Cell Proliferation : In various cell types, Ins(1,4,5)P3 signaling is linked to cell growth and proliferation.
Biological Functions
The biological activities of d-Myo-inositol-1,4,5-triphosphate can be categorized into several key functions:
1. Calcium Mobilization
Ins(1,4,5)P3 is integral in regulating intracellular calcium levels. Its binding to IP3Rs leads to rapid Ca²⁺ influx into the cytoplasm from the ER. This process is critical for numerous cellular functions including muscle contraction and neurotransmitter release.
2. Signal Transduction Pathways
Ins(1,4,5)P3 participates in various signaling cascades:
- Phosphoinositide Signaling : It is involved in pathways that modulate cell growth and differentiation.
- Apoptosis : Ins(1,4,5)P3 has been implicated in apoptotic pathways by influencing mitochondrial calcium levels.
3. Interaction with Other Molecules
Ins(1,4,5)P3 interacts with several proteins including:
- Protein Kinase C (PKC) : The presence of DAG alongside Ins(1,4,5)P3 activates PKC which further propagates signaling pathways.
- Calmodulin : Calcium released by Ins(1,4,5)P3 can bind to calmodulin influencing various downstream effects.
Case Studies
Several studies have explored the role of Ins(1,4,5)P3 in different biological contexts:
Case Study 1: Neuronal Signaling
Research demonstrated that Ins(1,4,5)P3 signaling is crucial for synaptic plasticity in hippocampal neurons. Mutations affecting IP3R function resulted in impaired long-term potentiation (LTP), a cellular mechanism underlying learning and memory .
Case Study 2: Cancer Biology
In cancer research, elevated levels of Ins(1,4,5)P3 have been associated with tumor growth and metastasis. Specifically, the A isoform of IP3K has been shown to be overexpressed in various tumor types . Targeting this pathway may provide therapeutic avenues for cancer treatment.
Research Findings
Recent studies have provided insights into the diverse roles of Ins(1,4,5)P3:
Propiedades
IUPAC Name |
[(1R,2S,3R,4R,5S,6R)-2,3,5-trihydroxy-4,6-diphosphonooxycyclohexyl] dihydrogen phosphate | |
---|---|---|
Source | PubChem | |
URL | https://pubchem.ncbi.nlm.nih.gov | |
Description | Data deposited in or computed by PubChem | |
InChI |
InChI=1S/C6H15O15P3/c7-1-2(8)5(20-23(13,14)15)6(21-24(16,17)18)3(9)4(1)19-22(10,11)12/h1-9H,(H2,10,11,12)(H2,13,14,15)(H2,16,17,18)/t1-,2+,3+,4-,5-,6-/m1/s1 | |
Source | PubChem | |
URL | https://pubchem.ncbi.nlm.nih.gov | |
Description | Data deposited in or computed by PubChem | |
InChI Key |
MMWCIQZXVOZEGG-XJTPDSDZSA-N | |
Source | PubChem | |
URL | https://pubchem.ncbi.nlm.nih.gov | |
Description | Data deposited in or computed by PubChem | |
Canonical SMILES |
C1(C(C(C(C(C1OP(=O)(O)O)O)OP(=O)(O)O)OP(=O)(O)O)O)O | |
Source | PubChem | |
URL | https://pubchem.ncbi.nlm.nih.gov | |
Description | Data deposited in or computed by PubChem | |
Isomeric SMILES |
[C@H]1([C@@H]([C@H]([C@@H]([C@H]([C@@H]1OP(=O)(O)O)O)OP(=O)(O)O)OP(=O)(O)O)O)O | |
Source | PubChem | |
URL | https://pubchem.ncbi.nlm.nih.gov | |
Description | Data deposited in or computed by PubChem | |
Molecular Formula |
C6H15O15P3 | |
Source | PubChem | |
URL | https://pubchem.ncbi.nlm.nih.gov | |
Description | Data deposited in or computed by PubChem | |
DSSTOX Substance ID |
DTXSID80893598 | |
Record name | Inositol 1,4,5-trisphosphate | |
Source | EPA DSSTox | |
URL | https://comptox.epa.gov/dashboard/DTXSID80893598 | |
Description | DSSTox provides a high quality public chemistry resource for supporting improved predictive toxicology. | |
Molecular Weight |
420.10 g/mol | |
Source | PubChem | |
URL | https://pubchem.ncbi.nlm.nih.gov | |
Description | Data deposited in or computed by PubChem | |
Physical Description |
Solid | |
Record name | myo-Inositol 1,4,5-trisphosphate | |
Source | Human Metabolome Database (HMDB) | |
URL | http://www.hmdb.ca/metabolites/HMDB0001498 | |
Description | The Human Metabolome Database (HMDB) is a freely available electronic database containing detailed information about small molecule metabolites found in the human body. | |
Explanation | HMDB is offered to the public as a freely available resource. Use and re-distribution of the data, in whole or in part, for commercial purposes requires explicit permission of the authors and explicit acknowledgment of the source material (HMDB) and the original publication (see the HMDB citing page). We ask that users who download significant portions of the database cite the HMDB paper in any resulting publications. | |
CAS No. |
85166-31-0 | |
Record name | Ins(1,4,5)P3 | |
Source | CAS Common Chemistry | |
URL | https://commonchemistry.cas.org/detail?cas_rn=85166-31-0 | |
Description | CAS Common Chemistry is an open community resource for accessing chemical information. Nearly 500,000 chemical substances from CAS REGISTRY cover areas of community interest, including common and frequently regulated chemicals, and those relevant to high school and undergraduate chemistry classes. This chemical information, curated by our expert scientists, is provided in alignment with our mission as a division of the American Chemical Society. | |
Explanation | The data from CAS Common Chemistry is provided under a CC-BY-NC 4.0 license, unless otherwise stated. | |
Record name | 1D-myo-inositol 1,4,5-trisphosphate | |
Source | DrugBank | |
URL | https://www.drugbank.ca/drugs/DB03401 | |
Description | The DrugBank database is a unique bioinformatics and cheminformatics resource that combines detailed drug (i.e. chemical, pharmacological and pharmaceutical) data with comprehensive drug target (i.e. sequence, structure, and pathway) information. | |
Explanation | Creative Common's Attribution-NonCommercial 4.0 International License (http://creativecommons.org/licenses/by-nc/4.0/legalcode) | |
Record name | Inositol 1,4,5-trisphosphate | |
Source | EPA DSSTox | |
URL | https://comptox.epa.gov/dashboard/DTXSID80893598 | |
Description | DSSTox provides a high quality public chemistry resource for supporting improved predictive toxicology. | |
Record name | INOSITOL 1,4,5-TRISPHOSPHATE | |
Source | FDA Global Substance Registration System (GSRS) | |
URL | https://gsrs.ncats.nih.gov/ginas/app/beta/substances/MU34XVK5NR | |
Description | The FDA Global Substance Registration System (GSRS) enables the efficient and accurate exchange of information on what substances are in regulated products. Instead of relying on names, which vary across regulatory domains, countries, and regions, the GSRS knowledge base makes it possible for substances to be defined by standardized, scientific descriptions. | |
Explanation | Unless otherwise noted, the contents of the FDA website (www.fda.gov), both text and graphics, are not copyrighted. They are in the public domain and may be republished, reprinted and otherwise used freely by anyone without the need to obtain permission from FDA. Credit to the U.S. Food and Drug Administration as the source is appreciated but not required. | |
Record name | myo-Inositol 1,4,5-trisphosphate | |
Source | Human Metabolome Database (HMDB) | |
URL | http://www.hmdb.ca/metabolites/HMDB0001498 | |
Description | The Human Metabolome Database (HMDB) is a freely available electronic database containing detailed information about small molecule metabolites found in the human body. | |
Explanation | HMDB is offered to the public as a freely available resource. Use and re-distribution of the data, in whole or in part, for commercial purposes requires explicit permission of the authors and explicit acknowledgment of the source material (HMDB) and the original publication (see the HMDB citing page). We ask that users who download significant portions of the database cite the HMDB paper in any resulting publications. | |
Retrosynthesis Analysis
AI-Powered Synthesis Planning: Our tool employs the Template_relevance Pistachio, Template_relevance Bkms_metabolic, Template_relevance Pistachio_ringbreaker, Template_relevance Reaxys, Template_relevance Reaxys_biocatalysis model, leveraging a vast database of chemical reactions to predict feasible synthetic routes.
One-Step Synthesis Focus: Specifically designed for one-step synthesis, it provides concise and direct routes for your target compounds, streamlining the synthesis process.
Accurate Predictions: Utilizing the extensive PISTACHIO, BKMS_METABOLIC, PISTACHIO_RINGBREAKER, REAXYS, REAXYS_BIOCATALYSIS database, our tool offers high-accuracy predictions, reflecting the latest in chemical research and data.
Strategy Settings
Precursor scoring | Relevance Heuristic |
---|---|
Min. plausibility | 0.01 |
Model | Template_relevance |
Template Set | Pistachio/Bkms_metabolic/Pistachio_ringbreaker/Reaxys/Reaxys_biocatalysis |
Top-N result to add to graph | 6 |
Feasible Synthetic Routes
Descargo de responsabilidad e información sobre productos de investigación in vitro
Tenga en cuenta que todos los artículos e información de productos presentados en BenchChem están destinados únicamente con fines informativos. Los productos disponibles para la compra en BenchChem están diseñados específicamente para estudios in vitro, que se realizan fuera de organismos vivos. Los estudios in vitro, derivados del término latino "in vidrio", involucran experimentos realizados en entornos de laboratorio controlados utilizando células o tejidos. Es importante tener en cuenta que estos productos no se clasifican como medicamentos y no han recibido la aprobación de la FDA para la prevención, tratamiento o cura de ninguna condición médica, dolencia o enfermedad. Debemos enfatizar que cualquier forma de introducción corporal de estos productos en humanos o animales está estrictamente prohibida por ley. Es esencial adherirse a estas pautas para garantizar el cumplimiento de los estándares legales y éticos en la investigación y experimentación.