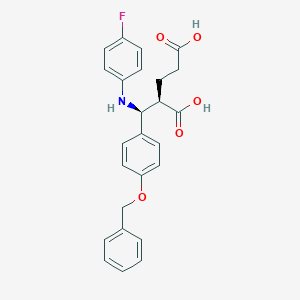
Ezetimibe Diacid Impurity
Descripción general
Descripción
Ezetimibe Diacid Impurity (CAS No. 1013025-04-1, molecular formula: C₂₅H₂₄FNO₅, molecular weight: 437.46 g/mol) is a process-related impurity observed during the synthesis of ezetimibe, a cholesterol absorption inhibitor. This impurity arises from hydrolytic or oxidative degradation pathways, leading to the introduction of carboxylic acid groups in the parent molecule . Regulatory guidelines, such as ICH Q3A, mandate strict control of such impurities (<0.10% for unknown impurities), necessitating robust analytical methods for quantification and structural characterization .
Métodos De Preparación
Synthetic Routes for Ezetimibe Diacid Impurity
Base-Catalyzed Hydrolysis of Ezetimibe
The most efficient method involves direct alkaline hydrolysis of Ezetimibe. As detailed in CN107474000A , the process comprises:
-
Reaction Setup : Ezetimibe is dissolved in polar aprotic solvents (e.g., tetrahydrofuran, N,N-dimethylformamide) or alcohols (methanol, ethanol).
-
Alkali Addition : Aqueous bases such as sodium bicarbonate (NaHCO₃), sodium carbonate (Na₂CO₃), or potassium carbonate (K₂CO₃) are introduced at pH 7.5–9.0.
-
Temperature Control : Reactions proceed at 0–30°C for 2–10 hours, monitored by TLC (dichloromethane:methanol = 20:1).
-
Workup : Acidification to pH 6–7 with HCl, followed by extraction with dichloromethane and chromatographic purification.
Key Advantages :
-
Yield : 62.3–71.8% (Table 1).
-
Purity : >95% by HPLC.
-
Scalability : No specialized equipment required.
Table 1: Yield and Purity Data for Base-Mediated Hydrolysis
Example | Solvent | Base | Temperature (°C) | Time (h) | Yield (%) | Purity (%) |
---|---|---|---|---|---|---|
1 | Tetrahydrofuran | NaHCO₃ | 5–10 | 4 | 71.8 | 96.1 |
2 | Methanol | NaHCO₃ | 20–30 | 5 | 68.2 | 95.8 |
3 | DMF | K₂CO₃ | 20–30 | 3 | 62.3 | 95.5 |
Historical Synthetic Approaches
Prior to CN107474000A, Cheng et al. (2013) reported a multi-step synthesis starting from a custom-synthesized intermediate :
-
Cyclization : Formation of the β-lactam core.
-
Deprotection : Removal of protective groups.
-
Reduction : Hydrogenation to yield the diacid.
Limitations :
-
Low Yield : 44% over three steps.
-
Complexity : Requires bespoke intermediates.
-
Safety Concerns : Hydrogenation poses explosion risks.
Optimization of Reaction Parameters
Solvent Selection
Polar solvents enhance alkali solubility and reaction homogeneity:
-
Tetrahydrofuran (THF) : Optimal for yield (71.8%) due to superior mixing with aqueous phases.
-
N,N-Dimethylformamide (DMF) : Lower yield (62.3%) attributed to viscosity-driven mass transfer limitations.
Base and pH Effects
-
NaHCO₃ (pH 8.0) : Higher yields (71.8%) vs. K₂CO₃ (62.3%) due to milder conditions preventing over-degradation.
-
pH <7.5 : Incomplete hydrolysis; pH >9 : Side reactions (e.g., decarboxylation).
Temperature and Kinetic Profile
-
0–10°C : Slower kinetics but higher purity (96.1%).
-
20–30°C : Faster reactions (3–5 hours) with slight purity trade-offs (95.5%).
Analytical Characterization
Chromatographic Purity Assessment
-
HPLC Conditions : C18 column, acetonitrile:water (70:30), 1.0 mL/min, UV detection at 254 nm.
-
Retention Time : 6.8 minutes (diacid) vs. 9.2 minutes (Ezetimibe).
Spectroscopic Confirmation
-
¹H NMR (400 MHz, DMSO-d₆) : δ 7.25 (d, 2H, Ar-F), 6.85 (d, 2H, Ar-OH), 5.12 (s, 1H, CH-OH).
-
MS (ESI+) : m/z 509.2 [M+H]⁺.
Comparative Analysis of Synthetic Methods
Table 2: Method Comparison for Diacid Impurity Synthesis
Parameter | CN107474000A | Cheng et al. (2013) |
---|---|---|
Steps | 1 | 3 |
Overall Yield | 62–72% | 44% |
Purity | >95% | 90–92% |
Scalability | High | Low |
Safety | Mild conditions | Hydrogenation hazards |
Industrial Applicability and Environmental Impact
The CN107474000A method is preferred for manufacturing due to:
Análisis De Reacciones Químicas
Ezetimibe Diacid Impurity undergoes various chemical reactions, including:
Oxidation: The compound can be oxidized using common oxidizing agents such as hydrogen peroxide or potassium permanganate.
Reduction: Reduction reactions can be carried out using reducing agents like sodium borohydride or lithium aluminum hydride.
Substitution: The impurity can undergo substitution reactions with reagents such as halogens or alkylating agents.
Hydrolysis: The compound can be hydrolyzed under acidic or basic conditions to yield different degradation products.
Common reagents and conditions used in these reactions include aqueous sodium hydroxide for hydrolysis, hydrogen peroxide for oxidation, and sodium borohydride for reduction. The major products formed from these reactions depend on the specific conditions and reagents used .
Aplicaciones Científicas De Investigación
Scientific Research Applications
Ezetimibe Diacid Impurity serves several important roles in scientific research:
Pharmaceutical Research
- Analytical Method Development : It is used to develop and validate analytical methods for detecting and quantifying impurities in Ezetimibe formulations. This ensures that pharmaceutical products meet regulatory standards .
- Impurity Profiling : The characterization of impurities, including this compound, is critical for understanding the stability and safety of drug formulations .
Stability Studies
- Degradation Pathways : Researchers study the stability of Ezetimibe under various conditions to understand its degradation pathways, which can inform storage and handling practices .
Toxicological Studies
- Safety Assessments : Toxicological research assesses the potential genotoxicity and overall safety profile of this compound, which is vital for evaluating its impact on human health .
Quality Control
- Reference Standards : The impurity is utilized as a reference standard in quality control laboratories to ensure the purity and safety of Ezetimibe products. This role is crucial in maintaining compliance with pharmacopoeial standards .
Case Studies
Stability testing guidelines from the International Conference on Harmonization (ICH) emphasize the importance of identifying degradation products like this compound. A study demonstrated that under alkaline conditions, significant degradation occurred, leading to the formation of this impurity. The findings highlighted the need for careful monitoring during drug formulation processes .
Mecanismo De Acción
The mechanism of action of Ezetimibe Diacid Impurity is not well-studied, as it is primarily a degradation product rather than an active pharmaceutical ingredient. its formation and presence in Ezetimibe formulations can affect the overall efficacy and safety of the drug. The impurity may interact with molecular targets and pathways involved in cholesterol absorption and metabolism, similar to Ezetimibe .
Comparación Con Compuestos Similares
Structural and Functional Similarities
Ezetimibe Diacid Impurity shares functional group characteristics with other diacid impurities in pharmaceuticals, such as:
- Pemetrexed 6-Oxo Diacid Impurity (CAS 193281-00-4): Features two carboxylic acid groups formed via oxidation of the parent drug .
- Levocabastine Diacid Impurity (CAS 2575516-82-2): Generated through hydrolysis of ester functionalities in levocabastine .
Key Structural Differences :
- Ezetimibe Diacid : Contains a fluorophenyl and hydroxyphenyl moiety, distinguishing it from pemetrexed and levocabastine derivatives.
- Pemetrexed 6-Oxo Diacid : Includes a pyrimidine ring, absent in ezetimibe-related impurities.
- Levocabastine Diacid: Possesses a benzazepine core, contrasting with ezetimibe’s azetidinone structure.
Formation Pathways
Compound | Formation Mechanism | Parent Drug Synthesis Step Affected |
---|---|---|
Ezetimibe Diacid | Hydrolysis/oxidation of ester groups | Late-stage intermediates |
Pemetrexed 6-Oxo Diacid | Oxidation of alcohol to carboxylic acid | Post-synthesis stability studies |
Desfluoro Ezetimibe* | Fluorine loss during synthesis | Early-stage intermediates |
*Desfluoro ezetimibe (CAS 302781-98-2) is another ezetimibe impurity, but it lacks diacid functionality .
Analytical Methodologies
This compound :
- HPLC : Retention time (RT) and relative retention time (RRT) are critical for identification. SynThink reports response factors (RF) and relative response factors (RRF) for quantification .
- Spectroscopy : NMR (¹H, ¹³C) and LC-MS confirm structural integrity .
Comparable Methods for Other Diacids :
- Pemetrexed 6-Oxo Diacid : Characterized via HNMR, IR, and mass spectrometry .
- Desfluoro Ezetimibe : Validated HPLC methods with limits of ≤0.15% and linearity (r² > 0.99) .
Regulatory and Control Strategies
*Assumed limit based on ICH Q3A guidelines for unknown impurities.
Actividad Biológica
Ezetimibe Diacid Impurity is a degradation product of Ezetimibe, a widely used lipid-lowering agent that functions primarily by inhibiting the absorption of cholesterol in the intestine. Understanding the biological activity of this compound is crucial for assessing its implications in pharmaceutical formulations, safety, and efficacy.
Chemical Background
Ezetimibe is known for its role in managing cholesterol levels by selectively inhibiting the Niemann-Pick C1-like 1 (NPC1L1) protein, which is essential for intestinal cholesterol absorption. The diacid impurity arises during the synthesis or storage of Ezetimibe and can influence both the pharmacological activity and safety profile of the drug.
Biological Activity Overview
The biological activities associated with this compound include:
- Inhibition of Cholesterol Absorption : Similar to Ezetimibe, the diacid impurity may also exhibit some degree of NPC1L1 inhibition, although its efficacy compared to the parent compound remains under investigation.
- Toxicological Profile : Research indicates that impurities like this compound require thorough toxicological assessments to evaluate potential genotoxic effects and overall safety in pharmaceutical applications .
Case Studies and Experimental Data
- Stability Studies : Stability testing has demonstrated that this compound can form under specific conditions, particularly through alkaline hydrolysis. The presence of this impurity necessitates rigorous quality control measures to ensure drug safety and efficacy .
- Impurity Profiling : A study highlighted the importance of impurity profiling in understanding how degradation products like this compound affect drug stability and patient outcomes. High-performance liquid chromatography (HPLC) methods have been employed to isolate and characterize these impurities, confirming their structures through various spectroscopic techniques .
- Toxicological Assessments : Preliminary studies suggest that while Ezetimibe is generally well-tolerated, its impurities, including the diacid form, may pose risks that warrant further investigation into their genotoxic potential. Such studies are critical for regulatory compliance and ensuring patient safety.
Data Tables
Parameter | Ezetimibe | This compound |
---|---|---|
Mechanism of Action | Inhibits NPC1L1 | Potential NPC1L1 inhibition |
Toxicological Profile | Generally well-tolerated | Requires further study |
Stability | Stable under normal conditions | Forms under alkaline conditions |
Analytical Methods | HPLC, LC-MS | HPLC, NMR |
Metabolic Pathways
Ezetimibe undergoes extensive metabolism primarily through glucuronidation facilitated by uridine 5′-diphosphate-glucuronosyltransferase (UGT) enzymes. The metabolic pathways for this compound are likely similar but require specific studies to elucidate any differences in pharmacokinetics or pharmacodynamics compared to the parent compound .
Q & A
Basic Research Questions
Q. What analytical methods are validated for quantifying Ezetimibe Diacid Impurity in drug formulations?
Reverse-phase high-performance liquid chromatography (HPLC) with UV detection is widely used, validated per ICH Q2(R1) guidelines. Key parameters include linearity (R² > 0.99995 across 0.1–10 µg/mL), detection limits (0.005–0.02 µg/mL), and specificity via spiking studies . Mobile phases often combine sodium buffer (pH 6.8) and acetonitrile with gradient elution to resolve co-eluting impurities .
Q. How should sample preparation be optimized to minimize diacid impurity degradation during analysis?
Solutions must be injected within 5 hours of preparation to prevent time-dependent increases in impurities like the tetrahydropyran (THP) compound, which can elevate relative standard deviation (RSD) to 1.82% for system precision . Use fresh diluents (e.g., solvent A:B = 4:6) and avoid prolonged storage to maintain stability .
Q. What regulatory thresholds apply to this compound in pharmaceuticals?
The USP mandates individual unknown impurities <0.20% and total impurities <0.60%. Batches must comply with these limits, validated via method accuracy (RSD ≤4.16%) and precision . ICH Q3A/B provides flexibility for qualification based on toxicological risk .
Q. How is method specificity confirmed for this compound in complex matrices?
Specificity is established by spiking drug substances with known impurities (e.g., desfluoro derivatives, ketone impurities) and demonstrating baseline separation. Residual plots and forced degradation studies (acid/base hydrolysis, oxidation) confirm no interference from excipients or degradation products .
Q. What are the critical validation parameters for impurity quantification methods?
ICH guidelines require:
- Linearity : 11 concentration levels spanning 0.01–1.00% of the active pharmaceutical ingredient (API).
- Accuracy : Recovery rates within 98–102% for spiked impurities.
- Precision : RSD ≤2% for repeatability and intermediate precision .
Advanced Research Questions
Q. How can co-eluting impurities structurally similar to Ezetimibe Diacid be resolved chromatographically?
Advanced gradient elution profiles (e.g., shifting from 70% to 10% aqueous phase over 30 minutes) improve resolution. Relative response factors (RRFs) and relative retention times (RRTs) are calculated to distinguish diacid impurities from analogs like the THP compound or ring-opened degradants .
Q. How should batch-to-batch variability in impurity profiles be investigated?
Variability arises from differences in API synthesis (e.g., Impurity D, an intermediate in ezetimibe synthesis). Root-cause analysis includes:
- Comparative HPLC profiling of batches.
- Assessing raw material quality and process parameters (e.g., temperature, catalysts) .
Q. What methodologies detect genotoxic diacid impurities at trace levels?
Reverse-phase HPLC coupled with mass spectrometry (LC-MS/MS) achieves detection limits <0.005 µg/mL for genotoxic impurities. Method validation includes forced degradation studies and genotoxicity assessments per ICH M7 .
Q. How do degradation pathways influence diacid impurity formation kinetics?
Stress testing (40°C/75% RH, oxidative conditions) reveals diacid impurities form via hydrolysis of ester groups. Kinetic modeling (e.g., Arrhenius plots) predicts shelf-life stability, while XRD and DSC confirm crystalline changes that accelerate degradation .
Q. What statistical tools address high variability in impurity stability data?
For impurities like the THP compound (RSD 1.82%), residual scatter plots and ANOVA identify outliers. Robustness testing evaluates method sensitivity to pH (±0.2), column temperature (±5°C), and flow rate (±10%) to refine acceptance criteria .
Propiedades
IUPAC Name |
(2R)-2-[(S)-(4-fluoroanilino)-(4-phenylmethoxyphenyl)methyl]pentanedioic acid | |
---|---|---|
Source | PubChem | |
URL | https://pubchem.ncbi.nlm.nih.gov | |
Description | Data deposited in or computed by PubChem | |
InChI |
InChI=1S/C25H24FNO5/c26-19-8-10-20(11-9-19)27-24(22(25(30)31)14-15-23(28)29)18-6-12-21(13-7-18)32-16-17-4-2-1-3-5-17/h1-13,22,24,27H,14-16H2,(H,28,29)(H,30,31)/t22-,24-/m1/s1 | |
Source | PubChem | |
URL | https://pubchem.ncbi.nlm.nih.gov | |
Description | Data deposited in or computed by PubChem | |
InChI Key |
WLAMIMDXLOJKMS-ISKFKSNPSA-N | |
Source | PubChem | |
URL | https://pubchem.ncbi.nlm.nih.gov | |
Description | Data deposited in or computed by PubChem | |
Canonical SMILES |
C1=CC=C(C=C1)COC2=CC=C(C=C2)C(C(CCC(=O)O)C(=O)O)NC3=CC=C(C=C3)F | |
Source | PubChem | |
URL | https://pubchem.ncbi.nlm.nih.gov | |
Description | Data deposited in or computed by PubChem | |
Isomeric SMILES |
C1=CC=C(C=C1)COC2=CC=C(C=C2)[C@H]([C@@H](CCC(=O)O)C(=O)O)NC3=CC=C(C=C3)F | |
Source | PubChem | |
URL | https://pubchem.ncbi.nlm.nih.gov | |
Description | Data deposited in or computed by PubChem | |
Molecular Formula |
C25H24FNO5 | |
Source | PubChem | |
URL | https://pubchem.ncbi.nlm.nih.gov | |
Description | Data deposited in or computed by PubChem | |
DSSTOX Substance ID |
DTXSID20647719 | |
Record name | (2R)-2-[(S)-[4-(Benzyloxy)phenyl](4-fluoroanilino)methyl]pentanedioic acid | |
Source | EPA DSSTox | |
URL | https://comptox.epa.gov/dashboard/DTXSID20647719 | |
Description | DSSTox provides a high quality public chemistry resource for supporting improved predictive toxicology. | |
Molecular Weight |
437.5 g/mol | |
Source | PubChem | |
URL | https://pubchem.ncbi.nlm.nih.gov | |
Description | Data deposited in or computed by PubChem | |
CAS No. |
1013025-04-1 | |
Record name | (2R)-2-[(S)-[4-(Benzyloxy)phenyl](4-fluoroanilino)methyl]pentanedioic acid | |
Source | EPA DSSTox | |
URL | https://comptox.epa.gov/dashboard/DTXSID20647719 | |
Description | DSSTox provides a high quality public chemistry resource for supporting improved predictive toxicology. | |
Retrosynthesis Analysis
AI-Powered Synthesis Planning: Our tool employs the Template_relevance Pistachio, Template_relevance Bkms_metabolic, Template_relevance Pistachio_ringbreaker, Template_relevance Reaxys, Template_relevance Reaxys_biocatalysis model, leveraging a vast database of chemical reactions to predict feasible synthetic routes.
One-Step Synthesis Focus: Specifically designed for one-step synthesis, it provides concise and direct routes for your target compounds, streamlining the synthesis process.
Accurate Predictions: Utilizing the extensive PISTACHIO, BKMS_METABOLIC, PISTACHIO_RINGBREAKER, REAXYS, REAXYS_BIOCATALYSIS database, our tool offers high-accuracy predictions, reflecting the latest in chemical research and data.
Strategy Settings
Precursor scoring | Relevance Heuristic |
---|---|
Min. plausibility | 0.01 |
Model | Template_relevance |
Template Set | Pistachio/Bkms_metabolic/Pistachio_ringbreaker/Reaxys/Reaxys_biocatalysis |
Top-N result to add to graph | 6 |
Feasible Synthetic Routes
Descargo de responsabilidad e información sobre productos de investigación in vitro
Tenga en cuenta que todos los artículos e información de productos presentados en BenchChem están destinados únicamente con fines informativos. Los productos disponibles para la compra en BenchChem están diseñados específicamente para estudios in vitro, que se realizan fuera de organismos vivos. Los estudios in vitro, derivados del término latino "in vidrio", involucran experimentos realizados en entornos de laboratorio controlados utilizando células o tejidos. Es importante tener en cuenta que estos productos no se clasifican como medicamentos y no han recibido la aprobación de la FDA para la prevención, tratamiento o cura de ninguna condición médica, dolencia o enfermedad. Debemos enfatizar que cualquier forma de introducción corporal de estos productos en humanos o animales está estrictamente prohibida por ley. Es esencial adherirse a estas pautas para garantizar el cumplimiento de los estándares legales y éticos en la investigación y experimentación.