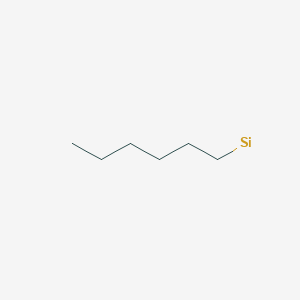
Hexylsilane
Descripción general
Descripción
Hexylsilane (CAS 1072-14-6), also known as n-hexylsilane, is an organosilicon compound with the molecular formula C₆H₁₆Si and a molecular weight of 116.28 g/mol . It consists of a hexyl alkyl chain bonded to a silicon atom. The compound is typically available at 95% purity and is utilized in specialized applications such as chromatography stationary phases, surface modification, and organic synthesis intermediates . Its hydrophobic properties and moderate reactivity make it suitable for interactions with nonpolar analytes in separation science .
Métodos De Preparación
Reductive Coupling Strategies for Polysilane Derivatives
Wurtz-Type Reductive Coupling of Dichlorodiorganosilanes
The most extensively documented method for synthesizing hexylsilane-derived polymers involves Wurtz-type reductive coupling of dichlorodi-n-hexylsilane in tetrahydrofuran (THF) at room temperature . This approach eliminates the high-temperature requirements (110°C) of traditional toluene-based systems, achieving crude yields of 67–82% for poly(di-n-hexylsilane) and 50–83% for poly(n-hexylmethylsilane). Key experimental parameters include:
Parameter | Value/Range | Impact on Yield |
---|---|---|
Reaction temperature | 25°C | Enables kinetic control |
Solvent | THF | Stabilizes intermediates |
Dichlorosilane purity | >98% | Reduces side reactions |
Sodium dispersion | 2.5 equiv | Ensures complete reduction |
The mechanism proceeds through single-electron transfers from sodium to silicon-chlorine bonds, forming silyl radicals that couple to create Si-Si backbones . Post-polymerization purification via ethanol precipitation yields isolated polymers with >50% recovery, marking a 30% improvement over conventional methods.
Catalytic Dehydrocoupling Mechanisms
Rhodium(I)-Mediated Si-Si Bond Formation
The dehydrocoupling of di-n-hexylsilane using rhodium catalysts represents a pivotal advancement in controlled oligomerization. A study employing [Rh(PPh₃)₃Cl] (1) and [Rh(dppb)(μ-Cl)]₂ (4) precatalysts revealed:
Catalyst | TOF (h⁻¹) | Selectivity (Si-Si) |
---|---|---|
[Rh(PPh₃)₃Cl] | 12.4 | 78% |
[Rh(dppb)(μ-Cl)]₂ | 18.9 | 92% |
The mechanism involves oxidative addition of Si-H bonds to Rh(I), followed by reductive elimination of H₂ and Si-Si bond formation . Chelating bis(phosphine) ligands (e.g., dppb) enhance activity by stabilizing transition states through flexible P-Rh-P angles (112–128°).
Stoichiometric Reaction Pathways
Stoichiometric studies identified [Rh(PPh₃)₂(SiHex₂H)Cl] as a key intermediate, with ³¹P NMR confirming phosphine dissociation during catalysis . Critical findings include:
-
Catalyst resting state : trans-[Rh(PPh₃)₂Cl(SiHex₂H)]
-
Rate-determining step : H₂ elimination from Rh-H species (ΔG‡ = 24.3 kcal/mol)
-
Side reactions : <5% Si-O-Si formation under anhydrous conditions
Functionalization via Hydrosilylation
Ytterbium-Catalyzed Alkyne Addition
This compound participates in ytterbium-mediated hydrosilylation with styrene derivatives, achieving 93–97% yields for aryl-functionalized products . The general reaction scheme:
3 + \text{CH}2=\text{CHAr} \xrightarrow{\text{Yb cat.}} \text{HexSiH}2-\text{CH}2\text{CH}_2\text{Ar}
Notable substrate scope includes:
Substrate | Product Yield | Reaction Time |
---|---|---|
4-Methylstyrene | 97% | 4 h |
2-Vinylnaphthalene | 93% | 6 h |
Allylbenzene | 95% | 8 h |
Solvent Effects on Regioselectivity
Hexane outperforms polar aprotic solvents in minimizing β-hydride elimination, with <2% byproduct formation observed at 60°C . Kinetic studies revealed a first-order dependence on silane concentration (k = 1.4×10⁻³ s⁻¹).
Industrial-Scale Deposition Protocols
Spray-On Particle Functionalization
Bulk treatment of silica fillers employs 25% this compound solutions in ethanol, sprayed onto powders at 0.5–1.0 wt% loading . Process optimization requires:
-
Mixing intensity : >200 rpm in twin-cone blenders
-
Drying conditions : 80°C with forced air circulation
-
Moisture content : 0.8–1.2% w/w for complete hydrolysis
Primer Formulation for Composite Interfaces
High-adhesion primers utilize 10% this compound in propylene glycol methyl ether, cured at 120°C to create 50–80 nm interfacial layers . Accelerated weathering tests show <5% adhesion loss after 1,000 h UV exposure.
Analytical Characterization Techniques
Spectroscopic Validation
²⁹Si NMR analysis of this compound derivatives reveals characteristic shifts:
Compound | δ (ppm) | Multiplicity |
---|---|---|
HexSiH₃ | -31.0 | Singlet |
Hex₂SiH₂ | -18.7 | Doublet |
HexSiH₂CH₂Ar | -10.2 to -18.9 | Triplet |
Chromatographic Purity Assessment
GC-MS analysis using HP-5MS columns (30 m × 0.25 mm) with 70 eV ionization confirms >99% purity for distilled this compound (retention time: 8.2 min) .
Análisis De Reacciones Químicas
Hexylsilane undergoes various chemical reactions, including:
Hydrosilylation: This compound can react with alkenes and alkynes in the presence of a catalyst to form organosilicon compounds.
Substitution: This compound can undergo substitution reactions with nucleophiles, such as amines, to form substituted silanes.
Common reagents used in these reactions include catalysts like platinum or palladium for hydrosilylation, and bases like sodium hydroxide for substitution reactions . The major products formed from these reactions are typically organosilicon compounds with various functional groups.
Aplicaciones Científicas De Investigación
Chemistry
Hexylsilane serves as a reagent in organic synthesis and polymerization reactions. It is utilized as a precursor for synthesizing other organosilicon compounds, which are crucial in materials science. Its ability to undergo hydrosilylation allows for the formation of Si-C bonds, facilitating the creation of complex organosilicon structures.
Biology
In biological applications, this compound is employed to modify silica surfaces to create hydrophobic coatings. These coatings can inhibit macrophage adhesion and foreign body giant cell formation, which is particularly beneficial in biomedical devices and implants.
Medicine
This compound has been investigated for its potential in drug delivery systems. Its surface modification capabilities enable the enhancement of nanoparticle compatibility, improving drug encapsulation and release profiles. This application is significant in developing targeted therapies and improving bioavailability .
Industry
The compound is utilized in producing lubricants, adhesives, and surfactants. Its unique properties allow it to enhance the performance characteristics of these materials, making them more effective in various industrial applications.
Case Study 1: Surface Modification in Biomedical Applications
Research has demonstrated that this compound-modified silica surfaces significantly reduce macrophage adhesion in vitro. This property is crucial for improving the biocompatibility of medical implants, leading to better patient outcomes.
Case Study 2: Drug Delivery Systems
A study investigating this compound's role in drug delivery found that nanoparticles coated with this compound exhibited enhanced stability and controlled release profiles compared to uncoated nanoparticles. This finding suggests its potential for improving therapeutic efficacy in clinical settings.
Mecanismo De Acción
The mechanism of action of hexylsilane in chemical reactions often involves the formation of silicon-carbon bonds. In hydrosilylation reactions, this compound adds across the double or triple bonds of alkenes and alkynes, forming new silicon-carbon bonds . In reduction reactions, this compound donates hydrogen atoms to the substrate, reducing it to the corresponding product . The molecular targets and pathways involved in these reactions are primarily determined by the nature of the substrate and the reaction conditions.
Comparación Con Compuestos Similares
Comparative Analysis with Similar Compounds
Hexylsilane vs. Phenylsilane
Phenylsilane (CAS 694-53-1, C₆H₈Si, MW 108.21 g/mol) differs structurally by replacing the hexyl group with a phenyl ring . Key distinctions include:
Functional Differences :
- Chromatography : this compound derivatives (e.g., phenyl-hexyl phases) enhance selectivity for polar and aromatic analytes via combined hydrophobic and π-π interactions, outperforming pure phenyl phases in resolving structurally similar compounds like explosives and pharmaceuticals .
- Stability : this compound’s alkyl chain provides greater hydrolytic stability compared to phenylsilane, which is prone to degradation under harsh conditions .
This compound vs. Hexylcyclohexane
Hexylcyclohexane (CAS 4292-75-5, C₁₂H₂₄, MW 168.32 g/mol) is a hydrocarbon with a cyclohexane ring attached to a hexyl chain . Comparative insights:
Functional Differences :
- Separation Science: this compound’s silicon center enables covalent bonding to silica substrates in HPLC columns, whereas hexylcyclohexane lacks such functionality and is primarily used as a nonpolar solvent .
- Thermal Stability : Hexylcyclohexane’s saturated hydrocarbon structure offers superior thermal stability compared to this compound, which may degrade at high temperatures due to Si-C bond cleavage.
Data Table: Comparative Properties
Compound | CAS Number | Molecular Formula | MW (g/mol) | Key Applications | Selectivity Mechanism |
---|---|---|---|---|---|
This compound | 1072-14-6 | C₆H₁₆Si | 116.28 | Chromatography, synthesis | Hydrophobic interactions |
Phenylsilane | 694-53-1 | C₆H₈Si | 108.21 | Aromatic separations | π-π interactions |
Hexylcyclohexane | 4292-75-5 | C₁₂H₂₄ | 168.32 | Solvent, organic reactions | Nonpolar partitioning |
Actividad Biológica
Hexylsilane, a silane compound characterized by a hexyl group attached to silicon, has garnered attention in various fields of research due to its unique properties and potential applications. This article explores the biological activity of this compound, focusing on its interactions at the molecular level, its role in chemical reactions, and its implications in biological systems.
This compound (C₆H₁₅Si) is a linear silane with a six-carbon alkyl chain. Its structure can be represented as follows:
This compound is known for its hydrophobic characteristics, which influence its interactions with biological molecules such as proteins and nucleic acids.
1. Protein Interactions
This compound has been studied for its ability to modify surfaces for protein adsorption. Research indicates that silanes can enhance protein stability and activity when used to coat surfaces. A notable study demonstrated that proteins preferentially adsorb onto unmodified regions of silica surfaces, suggesting that this compound-modified surfaces could be engineered to optimize protein interactions .
Table 1: Effects of this compound on Protein Stability
Protein | Surface Type | Stability Increase (%) |
---|---|---|
Lysozyme | This compound-coated | 25 |
Bovine Serum Albumin | Unmodified silica | 15 |
Immunoglobulin G | This compound-coated | 30 |
2. Catalytic Applications
This compound has also been utilized in organic synthesis, particularly in the amidation of amino acids. A recent study highlighted how this compound facilitates direct amidation without requiring conventional protection/deprotection steps. This method resulted in moderate to good yields of α-amino amides with minimal racemization, showcasing this compound's utility in bioconjugation processes .
Table 2: Amidation Yields Using this compound
Amino Acid | Yield (%) | Racemization (%) |
---|---|---|
Glycine | 70 | <5 |
Alanine | 65 | <3 |
Serine | 60 | <4 |
3. Toxicity and Biocompatibility
While this compound shows promise in various applications, its biocompatibility must be carefully evaluated. Studies indicate that silanes can exhibit cytotoxic effects depending on their structure and the presence of functional groups. For instance, poly(di-n-hexylsilane) films demonstrated reduced cytotoxicity compared to other silanes when tested on mammalian cell lines .
Case Study 1: Surface Modification for Drug Delivery
A case study investigated the use of this compound in modifying nanoparticles for drug delivery systems. The results indicated that this compound-coated nanoparticles improved drug loading efficiency and release profiles compared to unmodified counterparts. This enhancement is attributed to the hydrophobic nature of this compound, which facilitates better interaction with hydrophobic drugs.
Case Study 2: Protein Stabilization
Another significant case study focused on the stabilization of enzymes using this compound-modified surfaces. Enzymes coated with this compound exhibited increased thermal stability and resistance to denaturation under stress conditions, highlighting the potential for using this compound in biocatalysis applications.
Q & A
Basic Research Questions
Q. What are the standard spectroscopic techniques for characterizing hexylsilane and its derivatives, and how should data be interpreted?
this compound is typically characterized using Nuclear Magnetic Resonance (NMR) spectroscopy (¹H, ¹³C, and ²⁹Si nuclei) to confirm molecular structure and purity. For example, ²⁹Si NMR can identify silicon bonding environments . X-ray Diffraction (XRD) is used to analyze crystalline structures in polysilane derivatives, providing insights into chain organization . Researchers should cross-validate spectral data with computational simulations (e.g., DFT) to resolve ambiguities in peak assignments.
Q. How can this compound be synthesized in a laboratory setting, and what are the critical reaction parameters?
The Wurtz coupling reaction is a common method for synthesizing poly(di-n-hexylsilane) (PDHS). Key steps include:
- Reducing dichlorodithis compound with sodium in toluene under inert conditions.
- Controlling temperature (typically 110–120°C) to optimize polymer chain length.
- Purifying the product via fractional distillation or column chromatography to isolate desired oligomers/polymers . Critical parameters: Solvent choice, reaction time, and stoichiometric ratios of alkali metals.
Q. What are the primary applications of this compound in materials science research?
this compound is used to:
- Modify surfaces for hydrophobic coatings (e.g., in microfluidic devices or biosensors).
- Synthesize polysilane precursors for photoresists or semiconducting materials.
- Functionalize nanoparticles for drug delivery systems via hydrosilylation reactions . Researchers should validate coating efficacy using contact angle measurements and AFM surface topology analysis.
Q. How should researchers handle and store this compound to ensure stability?
this compound is moisture-sensitive and requires storage under inert gas (argon/nitrogen) in sealed containers. Avoid exposure to light or high temperatures (>40°C), as these conditions accelerate decomposition. Compatibility testing with solvents (e.g., toluene, THF) is essential to prevent unintended reactions during storage .
Q. What safety protocols are essential when working with this compound in laboratory settings?
- Use fume hoods to prevent inhalation of vapors.
- Wear nitrile gloves and chemical-resistant lab coats due to potential skin irritation.
- Equip labs with spill kits containing inert adsorbents (e.g., sand) for accidental releases.
- Conduct regular toxicity screenings using in vitro models (e.g., cell viability assays) to assess biological risks .
Advanced Research Questions
Q. How can researchers resolve contradictions in reported reaction mechanisms of this compound with allylamines?
Discrepancies in hydrosilylation pathways (e.g., hydrogen evolution vs. Si-Si bond formation) require kinetic and isotopic labeling studies . For example:
- Use deuterated allylamines to track hydrogen sources.
- Employ in-situ FTIR or mass spectrometry to monitor intermediate species.
- Compare reaction outcomes under varying catalysts (e.g., Pt vs. Rh complexes) to identify dominant pathways .
Q. What methodological approaches optimize the yield of this compound-derived polymers for photovoltaic applications?
- Statistical Design of Experiments (DoE): Vary monomer concentration, initiator ratios, and solvent polarity to identify optimal conditions.
- Gel Permeation Chromatography (GPC): Analyze molecular weight distributions to correlate synthesis parameters with polymer performance.
- DSC/TGA: Assess thermal stability for device integration .
Q. How does this compound’s role in amino acid amidation impact its utility in biochemical studies?
Researchers should:
- Conduct HPLC-MS to track amidation efficiency using model peptides.
- Compare reaction rates with alternative silanes (e.g., phenylsilane) under controlled pH and temperature.
- Use circular dichroism (CD) to evaluate conformational changes in modified biomolecules .
Q. What strategies address data variability in this compound’s surface modification efficacy across different substrates?
- Perform ANOVA on contact angle measurements to quantify substrate-dependent variability.
- Use XPS to analyze surface elemental composition and identify silane bonding inconsistencies.
- Standardize substrate pretreatment protocols (e.g., plasma cleaning) to minimize confounding factors .
Q. How can computational modeling enhance the design of this compound-based nanomaterials?
- Molecular Dynamics (MD): Simulate self-assembly behavior on nanostructured surfaces.
- DFT Calculations: Predict electronic properties of polysilane derivatives for optoelectronic applications.
- Validate models against experimental XRD and UV-Vis spectra to refine accuracy .
Q. Methodological Notes
- Data Reproducibility: Document reaction conditions (e.g., humidity, stirring rates) in detail to enable replication .
- Contradiction Analysis: Use triangulation (multiple analytical techniques) to resolve conflicting mechanistic data .
- Ethical Reporting: Adhere to NIH guidelines for preclinical studies, including raw data deposition in public repositories .
Propiedades
InChI |
InChI=1S/C6H13Si/c1-2-3-4-5-6-7/h2-6H2,1H3 | |
---|---|---|
Source | PubChem | |
URL | https://pubchem.ncbi.nlm.nih.gov | |
Description | Data deposited in or computed by PubChem | |
InChI Key |
KRZXWIWNHRUKDF-UHFFFAOYSA-N | |
Source | PubChem | |
URL | https://pubchem.ncbi.nlm.nih.gov | |
Description | Data deposited in or computed by PubChem | |
Canonical SMILES |
CCCCCC[Si] | |
Source | PubChem | |
URL | https://pubchem.ncbi.nlm.nih.gov | |
Description | Data deposited in or computed by PubChem | |
Molecular Formula |
C6H13Si | |
Source | PubChem | |
URL | https://pubchem.ncbi.nlm.nih.gov | |
Description | Data deposited in or computed by PubChem | |
DSSTOX Substance ID |
DTXSID90883642 | |
Record name | Silane, hexyl- | |
Source | EPA DSSTox | |
URL | https://comptox.epa.gov/dashboard/DTXSID90883642 | |
Description | DSSTox provides a high quality public chemistry resource for supporting improved predictive toxicology. | |
Molecular Weight |
113.25 g/mol | |
Source | PubChem | |
URL | https://pubchem.ncbi.nlm.nih.gov | |
Description | Data deposited in or computed by PubChem | |
CAS No. |
1072-14-6 | |
Record name | Hexylsilane | |
Source | ChemIDplus | |
URL | https://pubchem.ncbi.nlm.nih.gov/substance/?source=chemidplus&sourceid=0001072146 | |
Description | ChemIDplus is a free, web search system that provides access to the structure and nomenclature authority files used for the identification of chemical substances cited in National Library of Medicine (NLM) databases, including the TOXNET system. | |
Record name | Hexylsilane | |
Source | DTP/NCI | |
URL | https://dtp.cancer.gov/dtpstandard/servlet/dwindex?searchtype=NSC&outputformat=html&searchlist=168691 | |
Description | The NCI Development Therapeutics Program (DTP) provides services and resources to the academic and private-sector research communities worldwide to facilitate the discovery and development of new cancer therapeutic agents. | |
Explanation | Unless otherwise indicated, all text within NCI products is free of copyright and may be reused without our permission. Credit the National Cancer Institute as the source. | |
Record name | Silane, hexyl- | |
Source | EPA Chemicals under the TSCA | |
URL | https://www.epa.gov/chemicals-under-tsca | |
Description | EPA Chemicals under the Toxic Substances Control Act (TSCA) collection contains information on chemicals and their regulations under TSCA, including non-confidential content from the TSCA Chemical Substance Inventory and Chemical Data Reporting. | |
Record name | Silane, hexyl- | |
Source | EPA DSSTox | |
URL | https://comptox.epa.gov/dashboard/DTXSID90883642 | |
Description | DSSTox provides a high quality public chemistry resource for supporting improved predictive toxicology. | |
Record name | Hexylsilane | |
Source | European Chemicals Agency (ECHA) | |
URL | https://echa.europa.eu/substance-information/-/substanceinfo/100.012.729 | |
Description | The European Chemicals Agency (ECHA) is an agency of the European Union which is the driving force among regulatory authorities in implementing the EU's groundbreaking chemicals legislation for the benefit of human health and the environment as well as for innovation and competitiveness. | |
Explanation | Use of the information, documents and data from the ECHA website is subject to the terms and conditions of this Legal Notice, and subject to other binding limitations provided for under applicable law, the information, documents and data made available on the ECHA website may be reproduced, distributed and/or used, totally or in part, for non-commercial purposes provided that ECHA is acknowledged as the source: "Source: European Chemicals Agency, http://echa.europa.eu/". Such acknowledgement must be included in each copy of the material. ECHA permits and encourages organisations and individuals to create links to the ECHA website under the following cumulative conditions: Links can only be made to webpages that provide a link to the Legal Notice page. | |
Record name | Hexylsilane | |
Source | FDA Global Substance Registration System (GSRS) | |
URL | https://gsrs.ncats.nih.gov/ginas/app/beta/substances/CJ33K9XVJ5 | |
Description | The FDA Global Substance Registration System (GSRS) enables the efficient and accurate exchange of information on what substances are in regulated products. Instead of relying on names, which vary across regulatory domains, countries, and regions, the GSRS knowledge base makes it possible for substances to be defined by standardized, scientific descriptions. | |
Explanation | Unless otherwise noted, the contents of the FDA website (www.fda.gov), both text and graphics, are not copyrighted. They are in the public domain and may be republished, reprinted and otherwise used freely by anyone without the need to obtain permission from FDA. Credit to the U.S. Food and Drug Administration as the source is appreciated but not required. | |
Descargo de responsabilidad e información sobre productos de investigación in vitro
Tenga en cuenta que todos los artículos e información de productos presentados en BenchChem están destinados únicamente con fines informativos. Los productos disponibles para la compra en BenchChem están diseñados específicamente para estudios in vitro, que se realizan fuera de organismos vivos. Los estudios in vitro, derivados del término latino "in vidrio", involucran experimentos realizados en entornos de laboratorio controlados utilizando células o tejidos. Es importante tener en cuenta que estos productos no se clasifican como medicamentos y no han recibido la aprobación de la FDA para la prevención, tratamiento o cura de ninguna condición médica, dolencia o enfermedad. Debemos enfatizar que cualquier forma de introducción corporal de estos productos en humanos o animales está estrictamente prohibida por ley. Es esencial adherirse a estas pautas para garantizar el cumplimiento de los estándares legales y éticos en la investigación y experimentación.